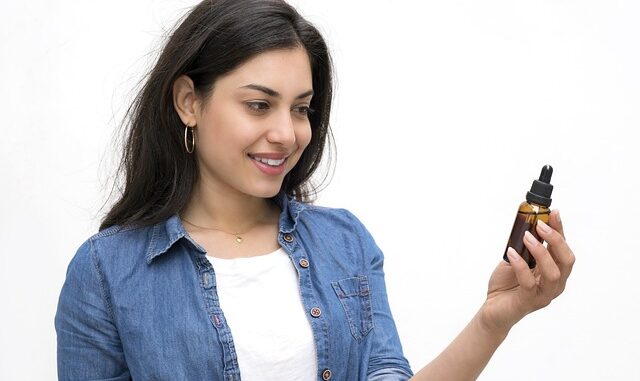
Abstract
The endocannabinoid system (ECS) is a complex and ubiquitous signaling network involved in regulating a diverse array of physiological processes, including pain modulation, inflammation, immune function, mood, appetite, and sleep. Cannabinoids, a class of compounds derived from the Cannabis sativa plant and also produced endogenously, interact with the ECS to exert their effects. This review provides a comprehensive overview of the ECS and its interactions with cannabinoids, encompassing a detailed exploration of specific cannabinoid types (e.g., THC, CBD, CBN), their individual and synergistic effects (the ‘entourage effect’), the challenges in determining optimal ratios for different conditions, and emerging insights into long-term effects. Furthermore, we examine the varying methods of cannabinoid administration, their pharmacokinetic and pharmacodynamic implications, and the current state of research on cannabinoid-based therapeutics. This review aims to offer an advanced perspective for experts in the field, highlighting both the therapeutic promise and the knowledge gaps that warrant further investigation.
Many thanks to our sponsor Maggie who helped us prepare this research report.
1. Introduction
The endocannabinoid system (ECS) has emerged as a crucial regulator of homeostasis across various organ systems. Its discovery and subsequent characterization have unveiled a complex network of endogenous ligands (endocannabinoids), receptors, and enzymes that govern the synthesis, transport, and degradation of these signaling molecules [1]. The ECS plays a critical role in maintaining physiological balance, influencing processes ranging from neuroplasticity and immune response to pain perception and metabolic regulation [2]. Disruption of ECS function has been implicated in numerous pathological conditions, including chronic pain, inflammation, neurodegenerative diseases, and mood disorders [3].
Cannabinoids, both endogenous and exogenous, interact with the ECS to modulate its activity. Cannabis sativa produces a vast array of cannabinoids, with Δ9-tetrahydrocannabinol (THC) and cannabidiol (CBD) being the most extensively studied. THC is primarily responsible for the psychoactive effects of cannabis, acting as a partial agonist at cannabinoid receptor type 1 (CB1) receptors, predominantly found in the brain and central nervous system. CBD, on the other hand, exhibits a more complex pharmacological profile, with low affinity for CB1 and CB2 receptors but interacting with a diverse range of other targets, including transient receptor potential (TRP) channels, G-protein coupled receptors (GPCRs), and enzymes [4]. This multifaceted mechanism of action underlies CBD’s diverse therapeutic potential, particularly in the context of pain, anxiety, and inflammation.
This review aims to provide an in-depth exploration of the ECS and its interactions with cannabinoids, moving beyond basic descriptions and delving into the nuanced mechanisms of action, synergistic effects, challenges in clinical application, and future directions in cannabinoid research. We will critically evaluate the existing literature, highlighting both the promising therapeutic applications and the gaps in our understanding that require further investigation.
Many thanks to our sponsor Maggie who helped us prepare this research report.
2. Components of the Endocannabinoid System
The ECS comprises three primary components: endocannabinoids, cannabinoid receptors, and enzymes involved in endocannabinoid metabolism [5]. Understanding each component is crucial for comprehending the complexities of cannabinoid signaling.
2.1 Endocannabinoids
The two major endocannabinoids are anandamide (AEA) and 2-arachidonoylglycerol (2-AG). AEA, discovered first, is a partial agonist at CB1 receptors, exhibiting lower efficacy compared to THC. It is synthesized from N-arachidonoyl phosphatidylethanolamine (NAPE) via a series of enzymatic steps. 2-AG, the most abundant endocannabinoid in the brain, is a full agonist at both CB1 and CB2 receptors. It is primarily synthesized from phosphatidylinositol bisphosphate (PIP2) via phospholipase C (PLC) and diacylglycerol lipase (DAGL) [6].
Both AEA and 2-AG are synthesized on demand, meaning they are not stored in vesicles but rather produced in response to neuronal activity or other stimuli. This ‘on-demand’ synthesis allows for rapid and localized modulation of synaptic transmission and cellular function. Furthermore, both endocannabinoids are rapidly degraded by enzymes, primarily fatty acid amide hydrolase (FAAH) for AEA and monoacylglycerol lipase (MAGL) for 2-AG [7]. The rapid synthesis and degradation ensure tight control over ECS signaling.
2.2 Cannabinoid Receptors
The two primary cannabinoid receptors are CB1 and CB2. CB1 receptors are predominantly expressed in the central nervous system (CNS), particularly in the cerebral cortex, hippocampus, basal ganglia, and cerebellum. They are also found in peripheral tissues, including the liver, gut, and adipose tissue. CB1 receptor activation in the brain mediates a wide range of effects, including psychoactivity, analgesia, appetite stimulation, and motor coordination [8].
CB2 receptors are primarily expressed in immune cells, such as macrophages, microglia, and B and T lymphocytes. They are also found in certain brain regions, although at lower levels than CB1 receptors. CB2 receptor activation modulates immune function, reducing inflammation and promoting immune cell migration [9]. The distinct expression patterns of CB1 and CB2 receptors contribute to the diverse effects of cannabinoids.
Beyond CB1 and CB2 receptors, cannabinoids interact with a variety of other receptors and ion channels, including TRP channels, peroxisome proliferator-activated receptors (PPARs), and GPR55 [10]. These interactions contribute to the pleiotropic effects of cannabinoids and further complicate our understanding of their mechanisms of action.
2.3 Enzymes of Endocannabinoid Metabolism
The enzymes involved in endocannabinoid synthesis and degradation play a critical role in regulating ECS activity. FAAH hydrolyzes AEA into arachidonic acid and ethanolamine, thereby terminating its signaling. MAGL hydrolyzes 2-AG into arachidonic acid and glycerol. Inhibition of FAAH or MAGL leads to increased levels of AEA or 2-AG, respectively, resulting in enhanced ECS signaling [11].
Other enzymes involved in endocannabinoid metabolism include NAPE-PLD (N-acylphosphatidylethanolamine phospholipase D), which catalyzes the synthesis of NAPE, the precursor of AEA, and DAGL, which catalyzes the synthesis of 2-AG. Targeting these enzymes represents a potential therapeutic strategy for modulating ECS activity and treating various conditions.
Many thanks to our sponsor Maggie who helped us prepare this research report.
3. Specific Cannabinoids and Their Effects
Cannabis sativa produces a diverse array of cannabinoids, each with its unique pharmacological profile. Understanding the specific effects of individual cannabinoids is crucial for developing targeted therapies.
3.1 Δ9-Tetrahydrocannabinol (THC)
THC is the primary psychoactive component of cannabis. It is a partial agonist at CB1 receptors, primarily responsible for the euphoric, analgesic, and anxiolytic effects of cannabis. However, at higher doses, THC can also induce anxiety, paranoia, and cognitive impairment [12]. The effects of THC are highly dose-dependent and vary significantly between individuals. Genetic factors, prior cannabis exposure, and the presence of other cannabinoids can all influence THC’s effects.
THC’s analgesic effects are mediated by both CB1 and CB2 receptors. CB1 receptor activation in the brain reduces pain perception, while CB2 receptor activation in immune cells reduces inflammation and pain associated with inflammation [13]. THC has been approved for the treatment of nausea and vomiting associated with chemotherapy and for appetite stimulation in patients with AIDS-related wasting syndrome. However, its psychoactive effects limit its widespread use.
3.2 Cannabidiol (CBD)
CBD is a non-psychoactive cannabinoid with a wide range of therapeutic potential. It has low affinity for CB1 and CB2 receptors but interacts with a variety of other targets, including TRP channels, PPARs, and GPCRs. CBD exhibits anti-inflammatory, analgesic, anxiolytic, and neuroprotective properties [14].
CBD’s analgesic effects are mediated by multiple mechanisms. It activates TRPV1 channels, which can desensitize pain pathways. It also inhibits the reuptake of anandamide, leading to increased levels of this endogenous cannabinoid. Furthermore, CBD reduces inflammation by modulating immune cell activity and suppressing the production of pro-inflammatory cytokines [15].
CBD has gained significant attention for its potential to treat various conditions, including chronic pain, anxiety disorders, epilepsy, and neurodegenerative diseases. Epidiolex, a CBD-based medication, has been approved for the treatment of certain types of epilepsy. However, more research is needed to fully understand CBD’s mechanisms of action and to determine its optimal dosage and efficacy for different conditions. Clinical trial data is still somewhat limited, and the quality of commercially available CBD products can vary significantly, posing challenges for both patients and clinicians.
3.3 Cannabinol (CBN)
CBN is a degradation product of THC, formed when THC is exposed to oxygen and heat. It is mildly psychoactive, with about one-tenth the potency of THC. CBN has been shown to have sedative effects and may contribute to the sleep-promoting properties of aged cannabis [16]. It also exhibits analgesic and anti-inflammatory properties.
CBN’s analgesic effects are thought to be mediated by activation of CB1 and CB2 receptors, as well as TRP channels. It has been shown to reduce pain in animal models of neuropathic pain and inflammatory pain [17]. However, more research is needed to fully understand CBN’s therapeutic potential and to determine its optimal dosage and efficacy.
3.4 Cannabigerol (CBG)
CBG is a non-psychoactive cannabinoid that is the precursor to THC, CBD, and CBC. It has been shown to have anti-inflammatory, analgesic, and neuroprotective properties. CBG interacts with a variety of targets, including CB1 and CB2 receptors, TRP channels, and α2-adrenergic receptors [18].
CBG’s analgesic effects are thought to be mediated by its interaction with TRP channels and α2-adrenergic receptors. It has been shown to reduce pain in animal models of inflammatory pain and neuropathic pain. CBG has also shown promise in the treatment of inflammatory bowel disease and glaucoma. However, clinical data on CBG are currently limited.
3.5 Other Cannabinoids
Numerous other cannabinoids are present in Cannabis sativa, including cannabichromene (CBC), cannabidivarin (CBDV), and tetrahydrocannabivarin (THCV). Each of these cannabinoids possesses unique pharmacological properties and may contribute to the overall therapeutic effects of cannabis. Research on these less-studied cannabinoids is ongoing, and their potential therapeutic applications are still being explored. In particular, THCV is attracting interest due to its seemingly paradoxical effects: it can act as both an agonist and antagonist at CB1 receptors, depending on the dose and context [19].
Many thanks to our sponsor Maggie who helped us prepare this research report.
4. The ‘Entourage Effect’: Synergistic Interactions
The ‘entourage effect’ refers to the synergistic interactions between different cannabinoids and other compounds in cannabis, such as terpenes and flavonoids. This concept suggests that the therapeutic effects of cannabis are greater than the sum of the individual effects of its components [20]. The entourage effect is a crucial consideration when developing cannabinoid-based therapies, as it highlights the importance of using whole-plant extracts or carefully formulated combinations of cannabinoids and other compounds.
Terpenes, the aromatic compounds found in cannabis, have been shown to interact with cannabinoids to modulate their effects. For example, myrcene, a common terpene in cannabis, enhances the analgesic effects of THC. Limonene, another terpene, has anxiolytic and antidepressant properties and may enhance the mood-elevating effects of cannabis [21]. Flavonoids, another class of compounds found in cannabis, also exhibit antioxidant and anti-inflammatory properties and may contribute to the overall therapeutic effects of cannabis.
Research on the entourage effect is still in its early stages, and more studies are needed to fully understand the complex interactions between cannabinoids and other compounds in cannabis. However, the existing evidence suggests that the entourage effect is a real phenomenon that should be considered when developing cannabinoid-based therapies. A major challenge is the standardization of these complex mixtures for clinical use.
Many thanks to our sponsor Maggie who helped us prepare this research report.
5. Optimal Ratios for Various Conditions
Determining the optimal ratios of cannabinoids for different conditions is a significant challenge in cannabinoid research. The effects of cannabinoids are highly dose-dependent and vary significantly between individuals. Furthermore, the optimal ratio of cannabinoids may depend on the specific condition being treated, as well as the individual’s genetic makeup, prior cannabis exposure, and other factors [22].
For example, some studies suggest that a 1:1 ratio of THC to CBD may be optimal for treating chronic pain, while other studies suggest that a higher ratio of CBD to THC may be more effective for treating anxiety disorders. However, these findings are not consistent across all studies, and more research is needed to determine the optimal ratios for different conditions. The subjective nature of pain and anxiety further complicates the determination of optimal ratios.
The development of personalized cannabinoid therapies, based on an individual’s genetic profile and other factors, may be necessary to optimize treatment outcomes. This approach would require sophisticated diagnostic tools and a deeper understanding of the complex interactions between cannabinoids and the ECS.
Many thanks to our sponsor Maggie who helped us prepare this research report.
6. Methods of Ingestion and Their Effects
The method of ingestion significantly affects the bioavailability, onset of action, and duration of effects of cannabinoids. Different methods of ingestion have distinct pharmacokinetic profiles, influencing the overall therapeutic experience [23].
6.1 Inhalation
Inhalation, via smoking or vaping, results in rapid absorption of cannabinoids into the bloodstream. The effects are typically felt within minutes, and the duration of effects is relatively short, lasting for 1-3 hours. Inhalation allows for precise titration of dosage, as the effects are felt quickly. However, smoking cannabis can expose individuals to harmful toxins and carcinogens. Vaping is generally considered a safer alternative, but the long-term effects of vaping are still unknown.
6.2 Oral Ingestion
Oral ingestion, via edibles or capsules, results in slower absorption of cannabinoids into the bloodstream. The effects are typically felt within 30-90 minutes, and the duration of effects is longer, lasting for 4-8 hours. Oral ingestion is convenient and discreet, but it is more difficult to titrate dosage due to the delayed onset of effects. Furthermore, oral ingestion results in higher levels of 11-hydroxy-THC, a more potent metabolite of THC, which can lead to more intense and prolonged psychoactive effects [24].
6.3 Sublingual Administration
Sublingual administration, via tinctures or lozenges placed under the tongue, results in faster absorption of cannabinoids into the bloodstream compared to oral ingestion. The effects are typically felt within 15-30 minutes, and the duration of effects is intermediate, lasting for 2-4 hours. Sublingual administration allows for relatively precise titration of dosage and avoids the first-pass metabolism that occurs with oral ingestion [25].
6.4 Topical Application
Topical application, via creams or lotions, results in localized effects without systemic absorption of cannabinoids. Topical application is used to treat localized pain, inflammation, and skin conditions. The effects are typically felt within minutes to hours, depending on the formulation and the condition being treated. Topical application avoids the psychoactive effects associated with other methods of ingestion.
6.5 Transdermal Administration
Transdermal administration, via patches, allows for sustained release of cannabinoids into the bloodstream. The effects are typically felt within hours, and the duration of effects can last for 24-72 hours. Transdermal administration provides a steady state of cannabinoids in the bloodstream, avoiding the peaks and valleys associated with other methods of ingestion. This can be particularly beneficial for managing chronic pain [26].
Many thanks to our sponsor Maggie who helped us prepare this research report.
7. Long-Term Effects of Cannabinoid Use
The long-term effects of cannabinoid use are a topic of ongoing research. While cannabis is generally considered safe, chronic use can have potential adverse effects, particularly on cognitive function, mental health, and cardiovascular health [27].
7.1 Cognitive Effects
Chronic cannabis use has been associated with impaired cognitive function, including deficits in attention, memory, and executive function. These effects are more pronounced in adolescents and young adults, whose brains are still developing. However, some studies suggest that these cognitive deficits may be reversible with abstinence [28]. The long-term cognitive effects of CBD are less well-studied, but preliminary evidence suggests that CBD may have neuroprotective effects and may not impair cognitive function.
7.2 Mental Health Effects
Chronic cannabis use has been associated with an increased risk of mental health problems, including anxiety, depression, and psychosis. However, the relationship between cannabis use and mental health is complex and may be bidirectional. Individuals with pre-existing mental health conditions may be more likely to use cannabis, and cannabis use may exacerbate these conditions. Furthermore, the specific cannabinoids used, the dosage, and the frequency of use can all influence the mental health effects of cannabis [29].
7.3 Cardiovascular Effects
Cannabis use can have acute cardiovascular effects, including increased heart rate and blood pressure. Chronic cannabis use has been associated with an increased risk of cardiovascular events, such as heart attack and stroke. However, the evidence is mixed, and more research is needed to fully understand the long-term cardiovascular effects of cannabis [30].
7.4 Other Long-Term Effects
Chronic cannabis use has also been associated with other potential adverse effects, including respiratory problems, impaired immune function, and reproductive problems. However, the evidence is limited, and more research is needed to confirm these associations.
The long-term effects of cannabinoid use are highly variable and depend on a variety of factors, including the specific cannabinoids used, the dosage, the frequency of use, and the individual’s genetic makeup and overall health. More research is needed to fully understand the long-term effects of cannabinoid use and to develop strategies to mitigate potential risks. Particular attention needs to be paid to the long-term effects of high-potency cannabis products and the increasingly prevalent use of cannabis among adolescents.
Many thanks to our sponsor Maggie who helped us prepare this research report.
8. Conclusion
The endocannabinoid system is a complex and ubiquitous signaling network that plays a critical role in maintaining physiological homeostasis. Cannabinoids, both endogenous and exogenous, interact with the ECS to modulate its activity, exerting a wide range of therapeutic effects. While significant progress has been made in understanding the ECS and the effects of cannabinoids, many challenges remain. Further research is needed to fully elucidate the mechanisms of action of individual cannabinoids, to understand the synergistic interactions between cannabinoids and other compounds (the entourage effect), and to determine the optimal ratios of cannabinoids for different conditions. Furthermore, more research is needed to fully understand the long-term effects of cannabinoid use and to develop strategies to mitigate potential risks.
As cannabinoid-based therapies become increasingly prevalent, it is crucial to base clinical decisions on rigorous scientific evidence. This requires well-designed clinical trials that address the complexities of cannabinoid pharmacology, including the entourage effect, individual variability, and the potential for long-term adverse effects. The development of personalized cannabinoid therapies, based on an individual’s genetic profile and other factors, may be necessary to optimize treatment outcomes. The future of cannabinoid research lies in a deeper understanding of the ECS and the development of targeted therapies that can harness the therapeutic potential of cannabinoids while minimizing potential risks. The current regulatory landscape, which varies widely across jurisdictions, poses significant challenges for conducting rigorous research and translating scientific findings into clinical practice.
Many thanks to our sponsor Maggie who helped us prepare this research report.
References
[1] Lu, H. C., & Mackie, K. (2016). An introduction to the endogenous cannabinoid system. Biological psychiatry, 79(7), 516-525.
[2] Zou, S., & Kumar, U. (2018). Cannabinoid receptors and the endocannabinoid system: signaling and function in the central nervous system. International journal of molecular sciences, 19(3), 833.
[3] Di Marzo, V., Bifulco, M., & De Petrocellis, L. (2004). The endocannabinoid system and its therapeutic exploitation. Nature Reviews Drug Discovery, 3(9), 771-784.
[4] El-Remessy, A. B., Khalil, I. E., Matragrano, J., Guzman, J., & Cutler, S. J. (2023). Targeting the endocannabinoid system in diabetic retinopathy. American Journal of Physiology-Endocrinology and Metabolism, 324(5), E391-E408.
[5] Piomelli, D. (2003). The molecular logic of endocannabinoid signalling. Nature Reviews Neuroscience, 4(10), 873-884.
[6] Kano, M., Ohno-Shosaku, T., Hashimotodani, Y., Uchigashima, M., & Watanabe, M. (2009). Endocannabinoid-mediated control of synaptic transmission. Physiological reviews, 89(1), 309-380.
[7] McPartland, J. M., Guy, G. W., Di Marzo, V., & Pertwee, R. G. (2014). Care and feeding of the endocannabinoid system: a systematic review of potential clinical interventions that upregulate the endocannabinoid system. PloS one, 9(3), e89566.
[8] Pertwee, R. G. (2008). The diverse CB1 and CB2 receptor pharmacology of three plant cannabinoids: Δ9-tetrahydrocannabinol, cannabidiol and Δ9-tetrahydrocannabivarin. British journal of pharmacology, 153(2), 199-215.
[9] Lu, T. K., & Anderson, D. C. (2017). The role of CB2 receptors in modulating immune function. Cannabis and cannabinoid research, 2(1), 28-36.
[10] De Petrocellis, L., Ligresti, A., Moriello, A. S., Allarà, M., Bisogno, T., Petrosino, S., … & Di Marzo, V. (2011). Effects of cannabinoids and cannabinoid-enriched Cannabis extracts on TRP channels and endocannabinoid metabolic enzymes. British journal of pharmacology, 163(7), 1479-1494.
[11] Kathuria, S., Gaetani, S., Fegley, D., Valiño, F., Duranti, A., Tontini, A., … & Piomelli, D. (2003). Modulation of the endocannabinoid system by fatty acid amides. Nature medicine, 9(1), 76-81.
[12] Volkow, N. D., Baler, R. D., Compton, W. M., & Weiss, S. R. B. (2014). Adverse health effects of marijuana use. New England Journal of Medicine, 370(23), 2219-2227.
[13] Elikashvili, I., & Alaverdashvili, M. (2021). The analgesic effect of cannabis-based medicines for chronic neuropathic pain: a systematic review and meta-analysis of randomized controlled trials. Journal of clinical medicine, 10(10), 2133.
[14] Blessing, E. M., Steenkamp, M. M., Manzanares, J., & Marmar, C. R. (2015). Cannabidiol as a potential treatment for anxiety disorders. Neurotherapeutics, 12(4), 825-836.
[15] Burstein, S. (2015). Cannabidiol (CBD) and its analogs: a review. Journal of clinical pharmacology, 55(3), 255-269.
[16] Farrimond, J. A., Whalley, B. J., & Williams, G. G. (2012). CBN and Δ9-THC-CBN interactions: sedation and activity in the mouse. Acta Pharmacologica Sinica, 33(9), 1175-1175.
[17] Russo, E. B. (2011). Taming THC: potential cannabis synergy and phytocannabinoid‐terpenoid entourage effects. British journal of pharmacology, 163(7), 1344-1364.
[18] Brierley, D. I., Samuels, J., Duncan, M., Whalley, B. J., & Williams, C. M. (2016). Cannabigerol is a novel, well-tolerated appetite stimulant in pre-satiated rats. Psychopharmacology, 233(19-20), 3603-3613.
[19] Pertwee, R. G. (2005). Pharmacological actions of cannabinoids. British journal of pharmacology, 146(6), 725-746.
[20] Russo, E. B. (2019). The case for the entourage effect and conventional breeding of clinical cannabis: no “strain,” no gain. Frontiers in plant science, 10, 145.
[21] Nuutinen, T. (2018). Medicinal properties of terpenes found in Cannabis sativa and Humulus lupulus. European journal of medicinal chemistry, 157, 198-228.
[22] MacCallum, C. A., & Russo, E. B. (2018). Practical considerations in medical cannabis administration and dosing. European journal of internal medicine, 49, 12-19.
[23] Huestis, M. A. (2007). Human cannabinoid pharmacokinetics. Chemistry & biodiversity, 4(8), 1770-1804.
[24] Perez-Reyes, M., Di Guiseppi, S., Ondrusek, G., Jeffcoat, A. R., & Cook, C. E. (1973). Free and protein-bound plasma cannabinoids in man. Clinical Pharmacology & Therapeutics, 14(4), 484-491.
[25] Abrams, D. I., Vizoso, H. P., Shade, S. B., Jay, C. A., Kelly, M. E., & Rowbotham, M. C. (2007). Vaporization as a smokeless cannabis delivery system: a pilot study. Clinical pharmacology & therapeutics, 82(5), 572-578.
[26] Stinchcomb, A. L., Valiveti, S., Hammell, D. C., & Aungst, B. J. (2004). Human skin permeation of Δ8-tetrahydrocannabinol, cannabidiol, and cannabinol. Journal of pharmaceutical sciences, 93(9), 2189-2200.
[27] Gage, S. H., Hickman, M., & Zammit, S. (2016). Association between cannabis and psychosis: epidemiologic evidence. Biological psychiatry, 79(7), 549-556.
[28] Gruber, S. A., Sagar, K. A., Dahlgren, M. K., Gonenc, A., & Pope Jr, H. G. (2014). Splendor in the grass? A pilot study assessing the impact of medical marijuana on executive function. Journal of cannabis therapeutics, 14(1), 1-23.
[29] Moore, T. H. M., Zammit, S., Ashok, A. H., Lewis, G., & MacCabe, J. H. (2007). Cannabis use and risk of psychotic or affective mental health outcomes: a systematic review. The Lancet, 370(9584), 319-329.
[30] Meier, D. G., Thomas, B. F., Mittleman, M. A., Auer, R., & Walsh, S. L. (2017). Cannabis and cardiovascular disease. Cardiology clinics, 35(1), 103-112.
Be the first to comment