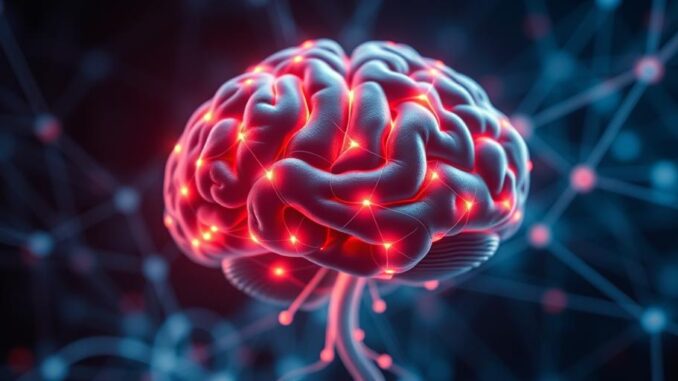
Abstract
Neuroplasticity, the brain’s inherent capacity to reorganize itself by forming new neural connections throughout life, is a fundamental mechanism underlying learning, memory, and adaptation to environmental changes. This report provides a comprehensive overview of neuroplasticity, encompassing its cellular and molecular mechanisms, various factors influencing its expression, and its implications for both normal brain function and neurological disorders. We delve into different forms of neuroplasticity, including synaptic plasticity, structural plasticity, and network plasticity, highlighting the distinct processes involved in each. Furthermore, we explore pharmacological and non-pharmacological interventions that can modulate neuroplasticity, with a particular focus on emerging therapeutic strategies for neurological and psychiatric conditions. The report also addresses the challenges and future directions in neuroplasticity research, emphasizing the need for a more nuanced understanding of its context-dependent nature and its complex interplay with genetic and environmental factors. We critically examine the potential of neuroplasticity-based therapies in addiction recovery and mental health treatment, specifically addressing the role of compounds like psilocybin in facilitating neural reorganization and promoting long-term behavioral changes. Finally, ethical considerations surrounding the manipulation of neuroplasticity are discussed, emphasizing the importance of responsible development and implementation of these powerful interventions.
Many thanks to our sponsor Maggie who helped us prepare this research report.
1. Introduction
Neuroplasticity, often described as the brain’s ability to change, is not merely a passive response to injury or disease but an active, dynamic process that shapes the nervous system throughout the lifespan. This capacity allows the brain to adapt to new experiences, recover from damage, and compensate for functional losses. The concept of neuroplasticity has undergone significant evolution since its initial conceptualization, shifting from the belief that the adult brain was largely immutable to the understanding that it possesses remarkable capacity for structural and functional reorganization. This paradigm shift has profound implications for our understanding of brain function in health and disease, as well as for the development of novel therapeutic interventions.
The historical roots of neuroplasticity can be traced back to the pioneering work of Santiago Ramón y Cajal, who proposed the “neuron doctrine” and emphasized the importance of synaptic connections in brain function. Donald Hebb’s concept of “neurons that fire together, wire together” further laid the foundation for understanding synaptic plasticity, the basis of learning and memory. Subsequent research has expanded our knowledge of neuroplasticity to include a broader range of mechanisms, including changes in dendritic spine morphology, axonal sprouting, and the formation of new neural circuits.
This report aims to provide a comprehensive overview of neuroplasticity, encompassing its underlying mechanisms, influencing factors, and therapeutic potential. We will explore the different forms of neuroplasticity, discuss the role of various pharmacological and non-pharmacological interventions in modulating its expression, and examine its implications for neurological and psychiatric disorders. Furthermore, we will critically evaluate the potential of neuroplasticity-based therapies for addiction recovery and mental health treatment, while addressing the ethical considerations surrounding the manipulation of this fundamental brain process.
Many thanks to our sponsor Maggie who helped us prepare this research report.
2. Mechanisms of Neuroplasticity
Neuroplasticity encompasses a wide range of cellular and molecular processes that contribute to the brain’s ability to reorganize itself. These processes can be broadly categorized into synaptic plasticity, structural plasticity, and network plasticity.
2.1 Synaptic Plasticity
Synaptic plasticity refers to changes in the strength and efficacy of synaptic connections between neurons. It is widely regarded as the cellular basis of learning and memory. The two major forms of synaptic plasticity are long-term potentiation (LTP) and long-term depression (LTD).
LTP is a persistent strengthening of synaptic connections, resulting from repeated high-frequency stimulation. It is characterized by an increase in the amplitude of excitatory postsynaptic potentials (EPSPs) and is thought to underlie the formation of new memories. The mechanisms underlying LTP involve the activation of NMDA receptors, influx of calcium ions, and activation of intracellular signaling cascades that lead to the insertion of AMPA receptors into the postsynaptic membrane [1].
LTD, conversely, is a persistent weakening of synaptic connections, resulting from low-frequency stimulation. It is characterized by a decrease in the amplitude of EPSPs and is thought to underlie the forgetting of irrelevant information. The mechanisms underlying LTD also involve the activation of NMDA receptors and calcium influx, but the specific signaling pathways activated differ from those involved in LTP [2].
Beyond LTP and LTD, other forms of synaptic plasticity include spike-timing-dependent plasticity (STDP), which depends on the precise timing of pre- and postsynaptic spikes, and metaplasticity, which refers to changes in the capacity for synaptic plasticity itself [3].
2.2 Structural Plasticity
Structural plasticity refers to changes in the physical structure of neurons, including alterations in dendritic spine morphology, axonal sprouting, and neurogenesis.
Dendritic spines are small protrusions on dendrites that serve as the postsynaptic sites for excitatory synapses. Changes in dendritic spine density and morphology are thought to play a crucial role in learning and memory. Experience-dependent changes in dendritic spines can occur rapidly, within minutes, and can involve the formation of new spines, the elimination of existing spines, and changes in spine size and shape [4].
Axonal sprouting refers to the growth of new axonal branches, allowing neurons to form new connections with other neurons. This process is particularly important for recovery from brain injury, as it allows surviving neurons to compensate for the loss of damaged neurons. Axonal sprouting is regulated by a variety of growth factors and guidance molecules [5].
Neurogenesis, the generation of new neurons, was once thought to be limited to early development. However, it is now known that neurogenesis continues throughout life in certain brain regions, particularly the hippocampus and the subventricular zone. Neurogenesis in the hippocampus is thought to play a role in learning, memory, and mood regulation [6].
2.3 Network Plasticity
Network plasticity refers to changes in the organization and function of neural circuits. These changes can involve alterations in the connectivity between different brain regions, as well as changes in the firing patterns of neurons within a network.
Network plasticity is essential for adapting to changing environmental demands and for recovering from brain injury. For example, after a stroke, the brain can reorganize itself to reroute neural signals around the damaged area, allowing patients to regain lost functions. Network plasticity is also involved in learning new skills, as the brain reorganizes itself to optimize performance [7].
Many thanks to our sponsor Maggie who helped us prepare this research report.
3. Factors Influencing Neuroplasticity
Neuroplasticity is not a fixed property of the brain but is rather a dynamic process that is influenced by a variety of factors, including genetics, experience, age, and disease state.
3.1 Genetic Factors
Genetic factors play a significant role in determining an individual’s capacity for neuroplasticity. Genes involved in synaptic transmission, neuronal growth, and neurotrophic signaling can influence the strength and efficiency of neuroplasticity [8]. Genetic polymorphisms in these genes can contribute to individual differences in learning, memory, and susceptibility to neurological disorders.
3.2 Experiential Factors
Experience is a powerful driver of neuroplasticity. Learning new skills, engaging in stimulating activities, and experiencing enriched environments can all promote neuroplasticity. Conversely, social isolation, chronic stress, and sensory deprivation can impair neuroplasticity [9]. The type and intensity of experience are critical determinants of the direction and magnitude of neuroplastic changes.
3.3 Age-Related Changes
Neuroplasticity is generally more pronounced in younger individuals, but it remains present throughout life. As we age, the brain’s capacity for neuroplasticity gradually declines, which may contribute to age-related cognitive decline. However, even in older adults, neuroplasticity can be enhanced through targeted interventions such as exercise and cognitive training [10].
3.4 Disease State
Neurological and psychiatric disorders can significantly impact neuroplasticity. For example, neurodegenerative diseases such as Alzheimer’s disease and Parkinson’s disease are characterized by a progressive loss of neurons and synaptic connections, which impairs neuroplasticity. Similarly, psychiatric disorders such as depression and schizophrenia are associated with alterations in brain structure and function that may reflect impaired neuroplasticity [11]. However, neuroplasticity can also play a role in recovery from these disorders, as the brain attempts to compensate for the damage or dysfunction.
Many thanks to our sponsor Maggie who helped us prepare this research report.
4. Modulation of Neuroplasticity
A variety of pharmacological and non-pharmacological interventions can modulate neuroplasticity. These interventions can be used to enhance neuroplasticity in healthy individuals to improve cognitive function, or to restore neuroplasticity in patients with neurological or psychiatric disorders.
4.1 Pharmacological Interventions
Several drugs have been shown to modulate neuroplasticity. These include:
- Neurotrophic factors: Brain-derived neurotrophic factor (BDNF) is a key regulator of neuroplasticity. Drugs that increase BDNF levels, such as antidepressants and exercise, can promote neuroplasticity [12].
- NMDA receptor modulators: NMDA receptors play a critical role in synaptic plasticity. Drugs that modulate NMDA receptor activity, such as memantine, can enhance LTP and improve cognitive function [13].
- Dopamine agonists: Dopamine is involved in reward and motivation, and dopamine agonists can enhance neuroplasticity in the reward circuitry, potentially contributing to addiction [14].
- Serotonergic psychedelics: Substances like psilocybin, found in psychedelic mushrooms, have demonstrated promise in promoting neuroplasticity. They are believed to induce neuroplasticity by stimulating the growth of new connections between neurons and by increasing the expression of neurotrophic factors. The specific mechanisms by which psilocybin facilitates these changes are complex and involve interactions with serotonin receptors, particularly the 5-HT2A receptor [15].
4.2 Non-Pharmacological Interventions
Several non-pharmacological interventions can also modulate neuroplasticity. These include:
- Exercise: Physical exercise has been shown to increase BDNF levels and promote neuroplasticity in the hippocampus and other brain regions. Exercise can improve cognitive function, reduce the risk of neurological disorders, and enhance recovery from brain injury [16].
- Cognitive training: Cognitive training involves practicing specific cognitive skills, such as memory or attention. This type of training can improve performance on the trained tasks and can also lead to broader improvements in cognitive function through the strengthening of relevant neural circuits [17].
- Brain stimulation: Non-invasive brain stimulation techniques, such as transcranial magnetic stimulation (TMS) and transcranial direct current stimulation (tDCS), can be used to modulate neuronal activity and promote neuroplasticity. These techniques have shown promise in treating a variety of neurological and psychiatric disorders [18].
- Sensory Enrichment: Providing enriched sensory environments can promote structural and functional plasticity. This may involve increasing exposure to visual, auditory, or tactile stimuli [19].
Many thanks to our sponsor Maggie who helped us prepare this research report.
5. Neuroplasticity in Addiction Recovery and Mental Health Treatment
Neuroplasticity plays a critical role in both the development and treatment of addiction and mental health disorders. Understanding the neuroplastic changes associated with these conditions is essential for developing effective interventions.
5.1 Addiction
Addiction is characterized by compulsive drug-seeking and drug-taking behavior, despite negative consequences. Chronic drug use leads to profound neuroplastic changes in the brain’s reward circuitry, particularly in the mesolimbic dopamine system. These changes include increased dopamine release in response to drug cues, decreased sensitivity to natural rewards, and impaired executive function.
These neuroplastic adaptations contribute to the development of craving, withdrawal symptoms, and relapse. Therapeutic interventions for addiction aim to reverse or counteract these maladaptive neuroplastic changes. Approaches such as cognitive behavioral therapy (CBT) and contingency management can promote the formation of new, adaptive neural circuits that support abstinence. Pharmacological interventions, such as naltrexone and buprenorphine, can also modulate neuroplasticity by reducing cravings and withdrawal symptoms [20].
As highlighted in the introduction, recent research explores the use of psychedelics, such as psilocybin, in addiction treatment. Psilocybin-assisted therapy is hypothesized to disrupt maladaptive neural patterns associated with addiction and promote the formation of new, healthier connections. The acute subjective experience induced by psilocybin may also facilitate insight and emotional processing, which can further support long-term recovery [21]. However, it is important to note that the use of psychedelics in addiction treatment is still in its early stages and requires further research to determine its efficacy and safety.
5.2 Mental Health
Mental health disorders, such as depression, anxiety, and post-traumatic stress disorder (PTSD), are associated with alterations in brain structure and function that may reflect impaired neuroplasticity. For example, depression is often associated with decreased hippocampal volume and reduced neurogenesis. Similarly, PTSD is associated with alterations in the amygdala and prefrontal cortex, which may contribute to heightened anxiety and impaired emotional regulation.
Therapeutic interventions for mental health disorders aim to restore or enhance neuroplasticity in these brain regions. Antidepressants, such as selective serotonin reuptake inhibitors (SSRIs), can increase BDNF levels and promote neurogenesis in the hippocampus. Psychotherapy, such as CBT and exposure therapy, can also promote neuroplasticity by helping patients to develop new coping mechanisms and to reprocess traumatic memories [22].
Brain stimulation techniques, such as TMS and tDCS, have shown promise in treating depression and PTSD by modulating neuronal activity and promoting neuroplasticity in the prefrontal cortex and other brain regions [23]. The use of psychedelics in treating these mental health conditions is also an area of active research.
Many thanks to our sponsor Maggie who helped us prepare this research report.
6. Challenges and Future Directions
Despite significant advances in our understanding of neuroplasticity, several challenges remain. These include:
- Context-dependent nature of neuroplasticity: Neuroplasticity is not a uniform process but is rather highly context-dependent. The specific mechanisms and outcomes of neuroplasticity depend on a variety of factors, including the brain region involved, the type of stimulus, and the individual’s genetic background and prior experience. Future research needs to focus on elucidating the specific contextual factors that determine the direction and magnitude of neuroplastic changes.
- Complexity of neural circuits: The brain is an incredibly complex network of interconnected neurons. Understanding how neuroplasticity shapes the organization and function of these circuits is a major challenge. Future research needs to employ advanced neuroimaging techniques and computational modeling to map the dynamic changes in neural circuits that occur during learning, adaptation, and recovery from injury.
- Long-term effects of neuroplasticity: While we have a good understanding of the short-term mechanisms of neuroplasticity, the long-term effects are less well understood. Future research needs to investigate the durability of neuroplastic changes and the factors that contribute to their maintenance or reversal.
- Translational challenges: Translating our understanding of neuroplasticity into effective therapeutic interventions remains a major challenge. Future research needs to focus on developing targeted interventions that can selectively modulate neuroplasticity in specific brain regions and circuits to treat neurological and psychiatric disorders.
Future research directions in neuroplasticity include:
- Developing novel neuroplasticity-enhancing drugs: There is a need for new drugs that can selectively enhance neuroplasticity in specific brain regions and circuits. These drugs could be used to treat a variety of neurological and psychiatric disorders, as well as to improve cognitive function in healthy individuals.
- Optimizing non-pharmacological interventions: There is a need for more research on how to optimize non-pharmacological interventions, such as exercise, cognitive training, and brain stimulation, to maximize their effects on neuroplasticity. This research could lead to the development of more effective rehabilitation strategies for patients with neurological disorders.
- Personalized neuroplasticity-based therapies: Future therapies could be tailored to the individual’s genetic background, prior experience, and specific brain pathology. This personalized approach could lead to more effective and targeted interventions for neurological and psychiatric disorders.
- Understanding the role of glial cells: Glial cells, such as astrocytes and microglia, play an increasingly recognized role in neuroplasticity. Further research is needed to elucidate the complex interactions between neurons and glial cells in the regulation of neuroplasticity.
Many thanks to our sponsor Maggie who helped us prepare this research report.
7. Ethical Considerations
The manipulation of neuroplasticity raises several ethical considerations. As we develop increasingly powerful interventions that can alter brain structure and function, it is important to consider the potential risks and benefits of these interventions, as well as their potential impact on individual autonomy and identity.
- Informed consent: Patients who are undergoing neuroplasticity-based therapies must be fully informed about the potential risks and benefits of the treatment, as well as any potential long-term consequences. They must also have the capacity to make an informed decision about whether or not to undergo the treatment.
- Autonomy and identity: Neuroplasticity-based therapies have the potential to alter an individual’s personality, beliefs, and values. It is important to ensure that these interventions are used in a way that respects individual autonomy and identity. Patients should have the right to refuse treatment, and they should be involved in decision-making about the goals of treatment.
- Justice and equity: Neuroplasticity-based therapies are likely to be expensive and may not be accessible to everyone. It is important to ensure that these therapies are distributed fairly and equitably, and that they are not used to exacerbate existing social inequalities.
- Potential for misuse: Neuroplasticity-enhancing drugs could potentially be misused for non-therapeutic purposes, such as cognitive enhancement or performance enhancement. It is important to develop policies and regulations to prevent the misuse of these drugs.
The responsible development and implementation of neuroplasticity-based therapies require careful consideration of these ethical issues. It is essential to engage in open and transparent dialogue about the potential risks and benefits of these interventions, and to develop ethical guidelines that ensure they are used in a way that promotes human well-being and respects individual rights.
Many thanks to our sponsor Maggie who helped us prepare this research report.
8. Conclusion
Neuroplasticity is a fundamental property of the brain that underlies learning, memory, adaptation, and recovery from injury. Understanding the mechanisms and modulation of neuroplasticity has profound implications for our understanding of brain function in health and disease. Pharmacological and non-pharmacological interventions that can modulate neuroplasticity offer promising therapeutic strategies for neurological and psychiatric disorders. However, further research is needed to address the challenges and complexities of neuroplasticity, and to ensure that these interventions are used responsibly and ethically.
Many thanks to our sponsor Maggie who helped us prepare this research report.
References
[1] Bliss, T. V. P., & Collingridge, G. L. (1993). A synaptic model of memory: long-term potentiation in the hippocampus. Nature, 361(6407), 31-39.
[2] Bear, M. F. (2003). Bidirectional synaptic plasticity: from theory to reality. Nature Neuroscience, 6(11), 1175-1186.
[3] Abraham, W. C. (2008). Metaplasticity: tuning the rules for synaptic plasticity. Trends in Neurosciences, 31(1), 8-17.
[4] Holtmaat, A., & Svoboda, K. (2009). Experience-dependent structural synaptic plasticity in the mammalian brain. Nature Reviews Neuroscience, 10(9), 647-659.
[5] Carmichael, S. T. (2005). Plasticity of cortical projections after stroke. Neuroscientist, 11(1), 75-84.
[6] Zhao, C., Deng, W., & Gage, F. H. (2008). Mechanisms and functional implications of adult neurogenesis. Cell, 132(4), 645-660.
[7] Dayan, E., & Cohen, L. G. (2011). Neuroplasticity subserving motor skill learning. Neuron, 72(3), 443-454.
[8] Hariri, A. R. (2009). The neurobiology of individual differences in complex behavioral traits. Annual Review of Neuroscience, 32, 225-247.
[9] Sale, A., Berardi, N., & Maffei, L. (2014). Environment and brain plasticity. Physiological Reviews, 94(2), 555-595.
[10] Kramer, A. F., Bherer, L., Colcombe, S. J., Dong, W., Greenough, W. T., & Kirk, I. J. (2004). Environmental influences on cognitive and brain plasticity during aging. The Journals of Gerontology Series B: Psychological Sciences and Social Sciences, 59(3), P94-P104.
[11] Duman, R. S. (2002). Synaptic plasticity and mood disorders. Biological Psychiatry, 52(8), 732-742.
[12] Duman, R. S., & Monteggia, L. M. (2006). A neurotrophic model for stress-related mood disorders. Biological Psychiatry, 59(12), 1116-1127.
[13] Lipton, S. A. (2006). Paradigm shift in neuroprotection by memantine and related drugs: change in NMDA receptor channel blockade from uncompetitive to open-channel block. Journal of Alzheimer’s Disease, 9(3 Suppl), 1-12.
[14] Volkow, N. D., Wang, G. J., Tomasi, D., & Baler, R. D. (2013). Addiction: hypothesis of disrupted motivation and drive. Trends in Cognitive Sciences, 17(3), 125-134.
[15] Ly, C., Greb, A. S., Cameron, L. P., et al. (2018). Psychedelics promote structural and functional plasticity in vitro and in vivo. Cell Reports, 23(11), 3170-3182.
[16] Cotman, C. W., Berchtold, N. C., & Christie, L. A. (2007). Exercise: a behavioral intervention to enhance brain health and plasticity. Trends in Neurosciences, 30(12), 617-622.
[17] Klingberg, T. (2010). Training and plasticity of working memory. Trends in Cognitive Sciences, 14(7), 317-324.
[18] Pascual-Leone, A., Tormos, J. M., Keenan, J., Tarazona, F., Cañete, C., & Catalá, M. D. (1998). Transcranial magnetic stimulation and neuroplasticity. Restorative Neurology and Neuroscience, 12(1), 55-59.
[19] Baroncelli, L., Sale, A., Viegi, A., & Maffei, L. (2010). Experience-dependent plasticity and visual acuity are modulated by an epigenetic mechanism. Brain, 133(9), 2686-2699.
[20] Koob, G. F., & Volkow, N. D. (2016). Neurobiology of addiction: a neurocircuitry analysis. The Lancet Psychiatry, 3(8), 760-773.
[21] Carhart-Harris, R. L., Leech, R., Erritzoe, D., Williams, T. M., Stone, J. M., Evans, J., … & Nutt, D. J. (2012). Neural correlates of the psychedelic state as determined by fMRI with psilocybin. Proceedings of the National Academy of Sciences, 109(6), 2138-2143.
[22] Beck, A. T. (2011). Cognitive therapy: basics and beyond. Guilford Press.
[23] O’Reardon, J. P., Solvason, H. B., Janicak, P. G., Sampson, S. M., Isenberg, K. E., Husain, M. M., … & Demitrack, M. A. (2007). Efficacy and safety of transcranial magnetic stimulation in the acute treatment of major depression: a multisite randomized controlled trial. Biological Psychiatry, 62(11), 1208-1216.
Be the first to comment