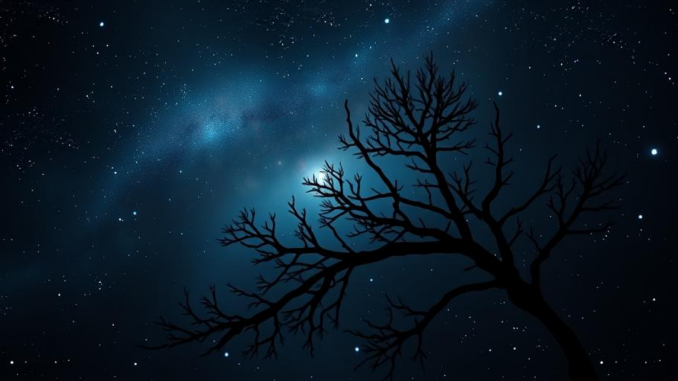
Abstract
Melatonin, the principal hormone of the pineal gland, plays a critical role in regulating circadian rhythms and influencing various physiological processes. This review provides a comprehensive overview of melatonin, encompassing its biosynthesis, regulatory mechanisms, chronobiological effects, and therapeutic applications. We delve into the intricate enzymatic pathways involved in melatonin synthesis, emphasizing the key regulatory enzymes and their susceptibility to environmental factors, particularly light. Furthermore, we explore the molecular mechanisms underlying melatonin’s chronobiological actions, focusing on its interaction with melatonin receptors (MT1 and MT2) and their downstream signaling pathways. The impact of light exposure, particularly blue light emitted from electronic devices, on melatonin suppression and circadian disruption is critically examined. Finally, we discuss the clinical use of melatonin supplements for various sleep disorders, including insomnia, jet lag, and shift work disorder, and explore the potential benefits and limitations of melatonin in treating other conditions such as neurodegenerative diseases, cancer, and mood disorders. Special emphasis is given to dosage considerations, efficacy data, safety profiles, and potential drug interactions.
Many thanks to our sponsor Maggie who helped us prepare this research report.
1. Introduction
Melatonin (N-acetyl-5-methoxytryptamine) is a pleiotropic hormone primarily synthesized and secreted by the pineal gland, a small endocrine structure located in the brain. It is synthesized from tryptophan through a series of enzymatic reactions. This hormone acts as a key regulator of the sleep-wake cycle and other circadian rhythms, which are approximately 24-hour oscillations in physiological processes that are entrained by environmental cues, primarily light-dark cycles. Beyond its role in circadian regulation, melatonin exhibits a wide array of physiological functions, including antioxidant activity, immune modulation, and regulation of reproductive hormones. The disruption of melatonin synthesis and signaling has been implicated in a variety of health problems, including sleep disorders, mood disorders, metabolic disturbances, and increased susceptibility to cancer. Therefore, a thorough understanding of melatonin’s biosynthesis, regulation, and physiological effects is crucial for developing effective therapeutic strategies targeting melatonin-related disorders.
Many thanks to our sponsor Maggie who helped us prepare this research report.
2. Biosynthesis and Regulation of Melatonin
2.1. Enzymatic Pathway
Melatonin biosynthesis is a multistep enzymatic process that begins with the essential amino acid tryptophan. The pathway involves four key enzymes: tryptophan hydroxylase (TPH), aromatic L-amino acid decarboxylase (AADC), serotonin N-acetyltransferase (AANAT), and hydroxyindole-O-methyltransferase (HIOMT). Tryptophan is first converted to 5-hydroxytryptophan (5-HTP) by TPH, the rate-limiting enzyme in serotonin synthesis. AADC then converts 5-HTP to serotonin (5-HT). AANAT, also known as arylalkylamine N-acetyltransferase, catalyzes the N-acetylation of serotonin to form N-acetylserotonin (NAS). Finally, HIOMT, also known as acetylserotonin O-methyltransferase (ASMT), catalyzes the O-methylation of NAS to form melatonin. [1]
2.2. Regulation of Melatonin Synthesis
The synthesis and secretion of melatonin are tightly regulated by the suprachiasmatic nucleus (SCN), the master circadian pacemaker located in the hypothalamus. The SCN receives direct input from the retina via the retinohypothalamic tract (RHT), allowing it to synchronize its activity to the external light-dark cycle. During darkness, the SCN sends signals through a multisynaptic pathway involving the paraventricular nucleus (PVN) and the superior cervical ganglion (SCG) to the pineal gland. This pathway ultimately leads to the release of norepinephrine from sympathetic nerve terminals in the pineal gland, which activates β-adrenergic receptors on pinealocytes.
Activation of β-adrenergic receptors stimulates the production of cyclic AMP (cAMP), which in turn activates protein kinase A (PKA). PKA phosphorylates and activates AANAT, the rate-limiting enzyme in melatonin synthesis. AANAT activity exhibits a robust circadian rhythm, with high activity during the night and low activity during the day. The daily rhythm of AANAT activity is primarily regulated by changes in both its transcription and its stability. In addition, HIOMT activity is relatively constant, indicating that AANAT is the key regulatory enzyme in melatonin synthesis.
2.3. Effects of Light on Melatonin Production
Light is the primary environmental cue that regulates melatonin synthesis. Exposure to light, particularly blue light (wavelengths between 460 and 480 nm), strongly suppresses melatonin production. Light exposure during the night shifts the circadian rhythm, potentially causing insomnia, as well as affecting alertness and mood. This suppression is mediated by intrinsically photosensitive retinal ganglion cells (ipRGCs) containing the photopigment melanopsin. ipRGCs project directly to the SCN, providing information about light exposure. When light is detected, the SCN inhibits the sympathetic pathway to the pineal gland, leading to a rapid decrease in AANAT activity and melatonin synthesis. The sensitivity of the melatonin system to light varies among individuals, with some individuals being more susceptible to light-induced melatonin suppression than others. [2]
Many thanks to our sponsor Maggie who helped us prepare this research report.
3. Chronobiological Effects of Melatonin
3.1. Melatonin Receptors and Signaling Pathways
Melatonin exerts its chronobiological effects by binding to two G protein-coupled receptors, MT1 and MT2, which are widely distributed throughout the body, including the brain, retina, cardiovascular system, and immune system. MT1 receptors are primarily located in the SCN and are involved in regulating sleep onset and promoting sleep. Activation of MT1 receptors inhibits neuronal firing and reduces the release of neurotransmitters that promote wakefulness. MT2 receptors are also expressed in the SCN and are involved in phase-shifting circadian rhythms. Activation of MT2 receptors can advance or delay the circadian clock, depending on the timing of melatonin administration.
Both MT1 and MT2 receptors couple to Gi/o proteins, inhibiting adenylyl cyclase and decreasing cAMP levels. In addition, MT1 receptors can activate phospholipase C (PLC), leading to an increase in intracellular calcium levels. MT2 receptors can also modulate the activity of mitogen-activated protein kinase (MAPK) signaling pathways. These diverse signaling pathways contribute to the wide range of physiological effects mediated by melatonin.
3.2. Regulation of Circadian Rhythms
Melatonin plays a critical role in synchronizing the body’s internal clock with the external environment. The rhythmic secretion of melatonin, with high levels during the night and low levels during the day, provides a strong circadian signal to various tissues and organs. Melatonin helps to regulate sleep-wake cycles, body temperature, hormone secretion, and other physiological processes that exhibit circadian rhythms. The timing of melatonin secretion is tightly controlled by the SCN, ensuring that these rhythms are properly aligned with the light-dark cycle.
3.3. Effects on Sleep
Melatonin promotes sleep by decreasing alertness and increasing sleep propensity. It exerts its sleep-promoting effects by binding to MT1 receptors in the SCN and other brain regions involved in sleep regulation. Melatonin also reduces sleep latency (the time it takes to fall asleep) and improves sleep quality. Furthermore, melatonin can counteract the alerting effects of light and other stimuli that disrupt sleep. The sleep-promoting effects of melatonin are particularly evident in individuals with delayed sleep phase disorder (DSPD), a circadian rhythm disorder characterized by a delayed sleep-wake cycle.
Many thanks to our sponsor Maggie who helped us prepare this research report.
4. Therapeutic Applications of Melatonin
4.1. Sleep Disorders
Melatonin supplements are widely used to treat various sleep disorders, including insomnia, jet lag, and shift work disorder. In insomnia, melatonin can help to reduce sleep latency, improve sleep quality, and increase total sleep time. The efficacy of melatonin in treating insomnia varies depending on the type and severity of the insomnia. Melatonin appears to be most effective in individuals with circadian rhythm sleep disorders, such as DSPD, and in older adults with age-related reductions in melatonin production.
Jet lag is a temporary sleep disorder that occurs when traveling across multiple time zones. Melatonin can help to alleviate jet lag symptoms by resynchronizing the circadian clock to the new time zone. The optimal timing of melatonin administration for jet lag depends on the direction of travel. In general, melatonin should be taken a few hours before bedtime in the new time zone for several days after arrival.
Shift work disorder is a sleep disorder that affects individuals who work irregular or rotating shifts. Melatonin can help to improve sleep quality and reduce daytime sleepiness in shift workers. However, the efficacy of melatonin in treating shift work disorder is somewhat inconsistent, and some studies have shown limited benefits.
4.2. Other Potential Applications
In addition to its use in treating sleep disorders, melatonin has shown promise in treating a variety of other conditions, including neurodegenerative diseases, cancer, and mood disorders. In neurodegenerative diseases such as Alzheimer’s disease and Parkinson’s disease, melatonin may protect against neuronal damage by reducing oxidative stress and inflammation. Melatonin has also been shown to have anti-cancer effects in vitro and in vivo, potentially inhibiting tumor growth and metastasis. In mood disorders such as depression and seasonal affective disorder (SAD), melatonin may help to regulate mood and improve sleep quality. However, further research is needed to confirm the efficacy and safety of melatonin in treating these conditions.
4.3. Dosage, Efficacy, Safety, and Drug Interactions
The optimal dosage of melatonin varies depending on the individual and the condition being treated. For sleep disorders, typical doses range from 0.3 mg to 5 mg taken 30 to 60 minutes before bedtime. Lower doses (e.g., 0.3-0.5 mg) are generally recommended for initial use, as they may be more effective and less likely to cause side effects than higher doses. The efficacy of melatonin depends on several factors, including the type and severity of the disorder, the timing of administration, and individual differences in melatonin sensitivity.
Melatonin is generally considered to be safe when taken at recommended doses. Common side effects include drowsiness, headache, dizziness, and nausea. However, some individuals may experience more severe side effects, such as vivid dreams, nightmares, and depression. Melatonin may interact with certain medications, including anticoagulants, antidepressants, and immunosuppressants. It is important to consult with a healthcare professional before taking melatonin supplements, especially if you are taking other medications or have any underlying health conditions.
Many thanks to our sponsor Maggie who helped us prepare this research report.
5. The Impact of Blue Light on Melatonin Production: A Detailed Analysis
The proliferation of digital devices such as smartphones, tablets, and computers has dramatically increased exposure to blue light, particularly in the evening hours. Blue light, with its shorter wavelengths, is particularly effective at suppressing melatonin production due to the spectral sensitivity of melanopsin in ipRGCs. The exposure to blue light directly impacts melatonin release. This section provides a more in-depth analysis of the mechanisms and consequences of blue light-induced melatonin suppression.
5.1. Spectral Sensitivity and Melanopsin
Melanopsin, the photopigment in ipRGCs, exhibits peak sensitivity to blue light around 480 nm. This sensitivity is significantly higher than that of traditional photoreceptors (rods and cones), making ipRGCs highly responsive to blue light. When blue light activates melanopsin, it triggers a signaling cascade that ultimately leads to the inhibition of melatonin synthesis in the pineal gland. The action spectrum of melanopsin closely mirrors the suppressive effect of light on melatonin, confirming its critical role in mediating this response. The level of blue light in electronic devices has increased over time, which greatly impacts the level of melatonin production.
5.2. Dose-Response Relationship
The extent of melatonin suppression is directly related to the intensity and duration of blue light exposure. Even relatively low levels of blue light can significantly reduce melatonin levels, especially when exposure occurs during the evening or nighttime hours. Studies have shown that as little as two hours of exposure to blue light from a digital device can suppress melatonin production by as much as 22%. [3] This dose-response relationship underscores the importance of minimizing blue light exposure in the evening.
5.3. Circadian Phase Shifting
In addition to suppressing melatonin production, blue light can also shift the circadian clock, delaying the onset of sleep and making it more difficult to wake up in the morning. This circadian phase-shifting effect is mediated by the SCN, which receives input from ipRGCs. Exposure to blue light in the evening signals to the SCN that it is still daytime, causing a delay in the body’s natural sleep-wake cycle. This can lead to chronic sleep deprivation and a range of other health problems.
5.4. Mitigation Strategies
Given the detrimental effects of blue light on melatonin production and circadian rhythms, several mitigation strategies have been developed to reduce blue light exposure. These include:
- Blue Light Filters: Many smartphones, tablets, and computers have built-in blue light filters that reduce the amount of blue light emitted from the screen. These filters can be activated manually or automatically scheduled to turn on in the evening.
- Blue Light-Blocking Glasses: These glasses have lenses that filter out blue light, reducing the amount of blue light that reaches the eyes. They are particularly useful for individuals who spend a lot of time using digital devices in the evening.
- Limiting Screen Time: Reducing screen time in the evening, especially in the hours before bedtime, can significantly reduce blue light exposure and improve sleep quality. It is recommended to avoid using digital devices for at least one hour before bed.
- Optimizing Lighting: Using warm-toned (red or orange) light bulbs in the evening can help to minimize blue light exposure and promote melatonin production. Avoiding bright, white light in the evening is also recommended.
5.5. Long-Term Consequences
The chronic suppression of melatonin by blue light exposure has been linked to a variety of long-term health problems, including:
- Sleep Disorders: Chronic exposure to blue light can disrupt the sleep-wake cycle and increase the risk of insomnia and other sleep disorders.
- Mood Disorders: Melatonin plays a role in regulating mood, and chronic suppression of melatonin has been linked to an increased risk of depression and other mood disorders.
- Metabolic Disorders: Disrupted circadian rhythms and suppressed melatonin production have been associated with an increased risk of obesity, diabetes, and other metabolic disorders.
- Cancer: Some studies have suggested that chronic melatonin suppression may increase the risk of certain types of cancer.
Many thanks to our sponsor Maggie who helped us prepare this research report.
6. Future Directions and Conclusion
Melatonin research continues to evolve, with ongoing investigations into its diverse physiological roles and therapeutic potential. Future research should focus on elucidating the precise mechanisms underlying melatonin’s actions, identifying novel melatonin receptor subtypes, and developing more effective melatonin analogs with improved bioavailability and receptor selectivity. The role of melatonin in the treatment of neurological and psychiatric disorders, including autism spectrum disorder, attention-deficit/hyperactivity disorder, and traumatic brain injury, warrants further investigation. Additionally, research into the potential benefits of melatonin in preventing and treating age-related diseases, such as Alzheimer’s disease and cardiovascular disease, is of great importance. Considering the increasing use of digital devices, further studies are needed to better understand the long-term health consequences of blue light exposure and to develop effective strategies for mitigating its effects on melatonin production and circadian rhythms. The interplay between melatonin and the gut microbiome is also an area of growing interest, as the gut microbiome can influence melatonin production and signaling. This relationship could have important implications for the treatment of sleep disorders and other conditions. The increasing availability of over-the-counter melatonin supplements necessitates careful evaluation of their quality, purity, and potential drug interactions. Overall, melatonin research holds great promise for improving human health and well-being.
In conclusion, melatonin is a versatile hormone with a wide range of physiological functions and therapeutic applications. Its role in regulating circadian rhythms and promoting sleep makes it a valuable tool for treating sleep disorders. However, it is important to use melatonin supplements judiciously and to be aware of their potential side effects and drug interactions. By continuing to investigate the complexities of melatonin’s biosynthesis, regulation, and actions, we can unlock its full therapeutic potential and improve the health and quality of life for individuals worldwide.
Many thanks to our sponsor Maggie who helped us prepare this research report.
References
[1] Hardeland, R. (2017). Melatonin: From molecular mechanisms to clinical practice. Springer.
[2] West, K. E., et al. (2011). Blue light from light-emitting diodes elicits a dose-dependent suppression of melatonin in humans. Journal of Applied Physiology, 110(3), 619-626.
[3] Gooley, J. J., et al. (2011). Exposure to room light before bedtime suppresses melatonin onset and shortens melatonin duration in humans. Journal of Clinical Endocrinology & Metabolism, 96(3), E463-E472.
[4] Auld, F., et al. (2017). Evidence for the efficacy of melatonin in the treatment of primary adult sleep disorders. Sleep Medicine Reviews, 34, 10-22.
[5] Costello, R. B., et al. (2014). The effectiveness of melatonin for promoting healthy sleep: a rapid evidence assessment of the literature. Journal of Clinical Sleep Medicine, 10(11), 1191-1200.
[6] Pandi-Perumal, S. R., et al. (2007). Melatonin: nature’s most versatile biological messenger. Journal of Pineal Research, 42(2), 141-166.
[7] Reiter, R. J., et al. (2014). Melatonin in reduction of oxidative stress and inflammation: significant clinical benefits. Journal of Investigative Medicine, 62(6), 936-942.
[8] Zhdanova, I. V., et al. (2001). Melatonin treatment for age-related insomnia. Journal of Clinical Endocrinology & Metabolism, 86(10), 4727-4730.
[9] Srinivasan, V., et al. (2010). Melatonin in mood disorders. Journal of Pineal Research, 48(4), 281-297.
[10] Buxton, O. M., Cain, S. W., O’Connor, S. P., Porter, J. H., Duffy, J. F., Wang, W., … & Czeisler, C. A. (2012). Acute phase shifting and suppression of melatonin secretion in response to monochromatic light exposure at fixed times. Journal of Biological Rhythms, 27(2), 145-155.
[11] Brainard, G. C., Hanifin, J. P., Greeson, J. M., Byrne, B., Glickman, G., James, M. C., … & Rollag, M. D. (2001). Action spectrum for melatonin regulation in humans: evidence for a novel photoreceptor. Journal of Neuroscience, 21(16), 6405-6412.
[12] Lockley, S. W., Brainard, G. C., & Czeisler, C. A. (2003). High sensitivity of the human circadian melatonin rhythm to resetting by short wavelength light. Journal of Clinical Endocrinology & Metabolism, 88(9), 4502-4505.
Be the first to comment