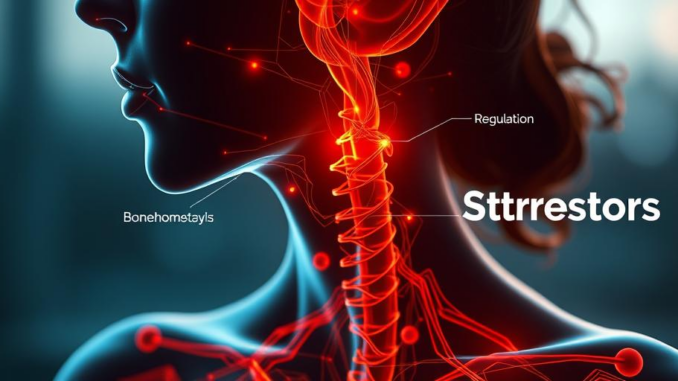
Abstract
Homeostasis, the maintenance of a stable internal environment, is a fundamental principle of physiology. This report delves into the complexities of homeostasis, exploring its underlying mechanisms, regulatory systems, and the challenges it faces in the context of internal and external perturbations. We examine the key components of homeostatic control, including feedback loops, sensors, integrators, and effectors, and their interplay in maintaining vital parameters such as temperature, pH, glucose levels, and electrolyte balance. Furthermore, we discuss the concept of allostasis, an adaptive process that enables the body to anticipate and respond to stressors, and contrast it with homeostasis. The report also addresses the limitations of the classical homeostatic model and the emergence of more nuanced perspectives that acknowledge the dynamic and context-dependent nature of physiological regulation. Finally, we explore the implications of homeostatic dysregulation in various pathological conditions, including but not limited to drug addiction, and highlight potential therapeutic strategies for restoring or modulating homeostatic balance.
Many thanks to our sponsor Maggie who helped us prepare this research report.
1. Introduction
The concept of homeostasis, coined by Walter Cannon, describes the body’s ability to maintain a relatively stable internal environment despite fluctuations in the external environment [1]. This dynamic equilibrium is crucial for the survival and optimal functioning of organisms. Claude Bernard, in the mid-19th century, first proposed the idea of a stable ‘milieu intérieur,’ recognizing that cells thrive within a constant internal environment. Cannon further expanded on this concept, emphasizing the coordinated physiological processes involved in maintaining stability [2].
Homeostasis is not a static state but rather a dynamic process involving continuous adjustments and regulations. It relies on intricate feedback loops, neural and hormonal signaling, and cellular mechanisms to counteract disturbances and maintain key physiological variables within a narrow range. Disruptions in homeostatic control can lead to various diseases and disorders, highlighting the importance of understanding its underlying mechanisms.
This report aims to provide a comprehensive overview of homeostasis, encompassing its fundamental principles, regulatory mechanisms, adaptive responses, and clinical implications. We will explore the role of various physiological systems in maintaining homeostasis, including the nervous, endocrine, and immune systems. Furthermore, we will discuss the concept of allostasis and its relationship to homeostasis, and examine the consequences of homeostatic dysregulation in disease. We will also touch upon emerging perspectives that challenge the classical view of homeostasis, emphasizing its dynamic and context-dependent nature.
Many thanks to our sponsor Maggie who helped us prepare this research report.
2. Fundamental Principles of Homeostasis
At its core, homeostasis operates through a series of interconnected components that detect deviations from the desired set point and initiate corrective actions. These components form the basis of feedback control loops, which are essential for maintaining stability. Key components include:
-
Sensors (Receptors): Specialized cells or structures that detect changes in the internal environment. These sensors are sensitive to specific variables, such as temperature, pH, pressure, and solute concentrations. Examples include thermoreceptors in the skin and hypothalamus, baroreceptors in blood vessels, and chemoreceptors in the brainstem.
-
Integrator (Control Center): The integrator receives information from the sensors and compares it to a predetermined set point, which represents the ideal value for the variable being regulated. If a deviation is detected, the integrator initiates a response to restore the variable to its set point. The brain, particularly the hypothalamus and brainstem, serves as the primary integrator for many homeostatic processes.
-
Effectors: These are the organs, tissues, or cells that carry out the response directed by the integrator. Effectors can include muscles, glands, and other specialized cells that alter their activity to counteract the disturbance. For example, sweat glands are effectors that help regulate body temperature, while the pancreas is an effector that releases hormones to regulate blood glucose levels.
2.1 Feedback Loops:
Feedback loops are the fundamental mechanisms by which homeostasis is achieved. There are two main types of feedback loops: negative and positive.
-
Negative Feedback Loops: These are the most common type of feedback loop in homeostatic control. They work to oppose deviations from the set point, restoring the variable to its desired range. When a variable deviates from its set point, the sensor detects the change and sends a signal to the integrator. The integrator then activates effectors that counteract the change, bringing the variable back towards the set point. For example, in the regulation of body temperature, if body temperature rises above the set point, thermoreceptors detect the change and send a signal to the hypothalamus. The hypothalamus then activates effectors, such as sweat glands and blood vessels in the skin, to promote heat loss and lower body temperature. Once body temperature returns to the set point, the negative feedback loop is deactivated.
-
Positive Feedback Loops: These loops amplify the initial deviation from the set point, leading to a greater change in the variable. Positive feedback loops are less common in homeostatic control because they can lead to instability and potentially harmful outcomes. However, they play important roles in certain physiological processes, such as blood clotting and childbirth. In blood clotting, the initial activation of clotting factors triggers a cascade of reactions that amplify the clotting process, leading to the formation of a blood clot. Similarly, during childbirth, uterine contractions stimulate the release of oxytocin, which further stimulates uterine contractions, leading to the delivery of the baby. Positive feedback loops are typically controlled by negative feedback loops or other regulatory mechanisms to prevent them from spiraling out of control.
2.2 Feedforward Control:
In addition to feedback control, feedforward control also plays a role in homeostasis. Feedforward control anticipates changes in the internal environment and initiates corrective actions before the variable deviates from its set point. This type of control relies on learned or predictive information about the environment. For example, the sight or smell of food can trigger the release of digestive enzymes in anticipation of food entering the digestive system. Feedforward control can help to minimize the impact of disturbances on the internal environment and maintain a more stable state.
Many thanks to our sponsor Maggie who helped us prepare this research report.
3. Key Homeostatically Regulated Parameters
Homeostasis encompasses the regulation of numerous physiological variables essential for survival. Some of the most critical parameters include:
-
Body Temperature: Maintaining a stable core body temperature is crucial for enzyme function and cellular processes. The body employs various mechanisms, including sweating, shivering, vasodilation, and vasoconstriction, to regulate heat loss and heat production. The hypothalamus acts as the primary thermoregulatory center [3].
-
Blood Glucose Levels: Maintaining a stable blood glucose concentration is essential for providing energy to cells. Insulin and glucagon, hormones secreted by the pancreas, regulate glucose uptake and release, respectively. Disruptions in glucose homeostasis can lead to diabetes mellitus [4].
-
Blood pH: Maintaining a narrow range of blood pH is critical for enzyme activity and protein structure. The body utilizes buffer systems, respiratory regulation, and renal regulation to control blood pH. Acidosis and alkalosis can result from imbalances in pH homeostasis [5].
-
Fluid and Electrolyte Balance: Maintaining proper fluid and electrolyte balance is essential for cell volume, nerve function, and muscle contraction. The kidneys play a central role in regulating fluid and electrolyte excretion, while hormones such as antidiuretic hormone (ADH) and aldosterone influence water and sodium reabsorption [6].
-
Blood Pressure: Maintaining adequate blood pressure is necessary for delivering oxygen and nutrients to tissues. The body employs various mechanisms, including baroreceptor reflexes, hormonal regulation (e.g., renin-angiotensin-aldosterone system), and autonomic nervous system control, to regulate blood pressure [7].
-
Oxygen and Carbon Dioxide Levels: Maintaining proper oxygen and carbon dioxide levels in the blood is essential for cellular respiration. The respiratory system regulates gas exchange in the lungs, while chemoreceptors in the brainstem and carotid arteries monitor blood oxygen and carbon dioxide levels and adjust ventilation accordingly [8].
Each of these parameters is tightly regulated by complex interactions between various physiological systems. Disruptions in the regulation of any of these parameters can have significant consequences for health and survival.
Many thanks to our sponsor Maggie who helped us prepare this research report.
4. Allostasis: Adaptation Beyond Homeostasis
While homeostasis emphasizes maintaining stability around a fixed set point, allostasis acknowledges the dynamic and adaptive nature of physiological regulation in response to stressors. Allostasis, a term coined by Sterling and Eyer, refers to the process by which the body actively adjusts its internal environment to meet anticipated demands [9]. Unlike homeostasis, which focuses on maintaining stability within a narrow range, allostasis allows for greater flexibility and adaptability in the face of changing conditions.
4.1 Allostatic Load:
When the demands of allostasis become chronic or excessive, the body experiences allostatic load. Allostatic load refers to the cumulative wear and tear on the body resulting from chronic overactivation or underactivation of allostatic systems. It can lead to various health problems, including cardiovascular disease, metabolic disorders, and mental health disorders [10].
4.2 Contrasting Homeostasis and Allostasis:
| Feature | Homeostasis | Allostasis |
| —————– | ———————————— | ——————————————– |
| Primary Focus | Maintaining stability | Adapting to anticipated demands |
| Set Point | Fixed | Variable, context-dependent |
| Regulatory Goal | Resisting change | Predicting and preparing for change |
| Time Scale | Short-term | Short- and long-term |
| Key Mechanisms | Negative feedback loops | Predictive regulation, behavioral adaptation |
| Potential Outcome | Stability or death | Adaptation, resilience, or allostatic load |
Allostasis expands upon the concept of homeostasis by recognizing that physiological regulation is not simply about maintaining stability but also about adapting to changing demands. It highlights the importance of considering the context in which physiological processes occur and the long-term consequences of chronic stress.
Many thanks to our sponsor Maggie who helped us prepare this research report.
5. Limitations of the Classical Homeostatic Model and Emerging Perspectives
The classical view of homeostasis, while foundational, has limitations in fully capturing the complexity of physiological regulation. Some criticisms include:
-
Overemphasis on Stability: The classical model tends to emphasize the importance of maintaining stability around a fixed set point, potentially overlooking the adaptive value of variability. Physiological variables often fluctuate naturally, and these fluctuations can be important for maintaining health.
-
Neglect of Context: The classical model often fails to consider the context in which physiological processes occur. Physiological regulation is highly dependent on environmental factors, social interactions, and individual experiences. Ignoring these contextual factors can lead to an incomplete understanding of homeostasis.
-
Oversimplification of Feedback Loops: The classical model often portrays feedback loops as simple, linear mechanisms. However, feedback loops are often complex and interconnected, with multiple inputs and outputs. This complexity can make it difficult to predict the effects of perturbations on the system.
Emerging perspectives challenge these limitations by emphasizing the dynamic, context-dependent, and multi-faceted nature of physiological regulation.
-
Network Physiology: This approach views the body as a complex network of interacting physiological systems. It emphasizes the importance of understanding how these systems communicate and coordinate with each other to maintain overall health [11].
-
Embodied Cognition: This perspective highlights the role of the body in shaping cognitive processes. It suggests that physiological states can influence perception, attention, and decision-making [12].
-
Predictive Processing: This framework proposes that the brain constantly generates predictions about the environment and updates these predictions based on sensory input. Physiological regulation is seen as a process of minimizing the discrepancy between predicted and actual states [13].
These emerging perspectives offer a more nuanced and comprehensive understanding of homeostasis and physiological regulation. They highlight the importance of considering the dynamic interactions between different physiological systems, the role of context in shaping physiological responses, and the predictive nature of brain function.
Many thanks to our sponsor Maggie who helped us prepare this research report.
6. Homeostatic Dysregulation in Disease
Disruptions in homeostatic control are implicated in a wide range of diseases and disorders. These disruptions can result from genetic mutations, environmental factors, or lifestyle choices. Some examples of diseases associated with homeostatic dysregulation include:
-
Diabetes Mellitus: This metabolic disorder is characterized by impaired glucose homeostasis, resulting from either insufficient insulin production (Type 1) or insulin resistance (Type 2) [4].
-
Hypertension: This cardiovascular disorder is characterized by elevated blood pressure, resulting from dysregulation of blood pressure control mechanisms [7].
-
Chronic Kidney Disease: This condition is characterized by impaired kidney function, leading to disruptions in fluid and electrolyte balance, as well as acid-base balance [6].
-
Neurodegenerative Diseases: Diseases such as Alzheimer’s and Parkinson’s are associated with disruptions in neuronal homeostasis, including imbalances in neurotransmitter levels and impaired protein clearance [14].
-
Drug Addiction: Chronic drug use can disrupt brain homeostasis, particularly in reward pathways, leading to dependence and addiction. This involves alterations in neurotransmitter systems, receptor sensitivity, and neuronal circuitry [15]. The article to which this report is an answer refers to this specific case.
The specific mechanisms by which homeostatic dysregulation contributes to disease vary depending on the disorder. However, common themes include impaired feedback control, altered set points, and damage to regulatory organs or tissues. Understanding the role of homeostatic dysregulation in disease is crucial for developing effective therapeutic strategies.
Many thanks to our sponsor Maggie who helped us prepare this research report.
7. Therapeutic Strategies for Restoring or Modulating Homeostasis
Therapeutic interventions aimed at restoring or modulating homeostasis are central to the treatment of many diseases. These interventions can target specific components of the homeostatic control system or address the underlying causes of dysregulation. Some examples of therapeutic strategies include:
-
Pharmacological Interventions: Medications can be used to directly manipulate physiological variables, such as blood glucose levels (e.g., insulin), blood pressure (e.g., antihypertensives), and electrolyte balance (e.g., diuretics). Other medications can target specific receptors or enzymes involved in homeostatic control.
-
Lifestyle Modifications: Changes in diet, exercise, and stress management can have a profound impact on homeostasis. For example, regular exercise can improve insulin sensitivity and blood glucose control, while a healthy diet can help to maintain electrolyte balance and blood pressure.
-
Medical Devices: Medical devices can be used to assist or replace failing organs or tissues involved in homeostatic regulation. Examples include dialysis machines for kidney failure, pacemakers for heart arrhythmias, and insulin pumps for diabetes.
-
Gene Therapy: In some cases, genetic mutations can contribute to homeostatic dysregulation. Gene therapy aims to correct these mutations by introducing functional copies of the affected gene into the patient’s cells [16].
-
Neurofeedback and Biofeedback: These techniques allow individuals to gain conscious control over physiological processes, such as heart rate, brainwave activity, and muscle tension. This can help to improve self-regulation and restore homeostatic balance [17].
The choice of therapeutic strategy depends on the specific disease and the underlying mechanisms of homeostatic dysregulation. In many cases, a combination of approaches may be necessary to achieve optimal outcomes. Furthermore, personalized medicine approaches, which take into account individual genetic and environmental factors, are increasingly being used to tailor therapeutic interventions to the specific needs of each patient.
Many thanks to our sponsor Maggie who helped us prepare this research report.
8. Conclusion
Homeostasis is a fundamental principle of physiology that underlies the body’s ability to maintain a stable internal environment. This report has explored the key components of homeostatic control, including feedback loops, sensors, integrators, and effectors, and their interplay in regulating vital physiological variables. We have also discussed the concept of allostasis and its relationship to homeostasis, and examined the limitations of the classical homeostatic model and the emergence of more nuanced perspectives. Furthermore, we have highlighted the implications of homeostatic dysregulation in various diseases and disorders and explored potential therapeutic strategies for restoring or modulating homeostatic balance.
While significant progress has been made in understanding homeostasis, many challenges remain. Future research should focus on elucidating the complex interactions between different physiological systems, the role of context in shaping physiological responses, and the mechanisms by which chronic stress leads to allostatic load. Furthermore, there is a need for developing more effective therapeutic strategies that target the underlying causes of homeostatic dysregulation and promote personalized medicine approaches. By continuing to advance our understanding of homeostasis, we can improve the prevention and treatment of a wide range of diseases and disorders and enhance human health and well-being.
Many thanks to our sponsor Maggie who helped us prepare this research report.
References
[1] Cannon, W. B. (1929). Organization for physiological homeostasis. Physiological Reviews, 9(3), 399-431.
[2] Bernard, C. (1865). An introduction to the study of experimental medicine. Paris: Baillière.
[3] Morrison, S. F. (2016). Central neural pathways for thermoregulation. Frontiers in Bioscience, 21, 1907-1919.
[4] DeFronzo, R. A. (2009). From the triumvirate to the ominous octet: a new paradigm for the treatment of type 2 diabetes mellitus. Diabetes, 58(4), 773-795.
[5] Hamm, L. L., Nakhoul, N., & Hering-Smith, K. S. (2015). Acid-base homeostasis. Clinical Journal of the American Society of Nephrology, 10(12), 2232-2242.
[6] Palmer, B. F., & Clegg, D. J. (2016). Physiology and pathophysiology of body fluid homeostasis. Advances in Physiology Education, 40(4), 462-474.
[7] Hall, J. E., & Guyton, A. C. (2006). Guyton and Hall textbook of medical physiology. Elsevier Saunders.
[8] Prabhakar, N. R., & Semenza, G. L. (2015). Oxygen sensing and homeostasis. Physiological Reviews, 95(1), 1-43.
[9] Sterling, P., & Eyer, J. (1988). Allostasis: a new paradigm to explain arousal pathology. In S. Fisher & J. Reason (Eds.), Handbook of life stress, cognition and health (pp. 629-649). John Wiley & Sons.
[10] McEwen, B. S. (1998). Protective and damaging effects of stress mediators. New England Journal of Medicine, 338(3), 171-179.
[11] Ivanov, P. C., Bartsch, R. P., & Stanley, H. E. (2017). Network physiology: Understanding organ systems interactions. American Journal of Physiology-Regulatory, Integrative and Comparative Physiology, 312(6), R1037-R1061.
[12] Barrett, L. F. (2017). How emotions are made: The secret life of the brain. Houghton Mifflin Harcourt.
[13] Clark, A. (2013). Whatever next? Predictive brains, situated agents, and the future of cognitive science. Behavioral and Brain Sciences, 36(3), 181-204.
[14] Mattson, M. P. (2008). Apoptosis in neurodegenerative disorders. Nature Reviews Neuroscience, 1(1), 120-129.
[15] Koob, G. F., & Volkow, N. D. (2016). Neurobiology of addiction: a neurocircuitry analysis. The Lancet Psychiatry, 3(8), 760-773.
[16] Anderson, W. F. (1998). Human gene therapy. Nature, 392(6679 Suppl), 25-30.
[17] Hammond, D. C. (2011). What is neurofeedback? Journal of Neurotherapy, 15(4), 305-336.
Be the first to comment