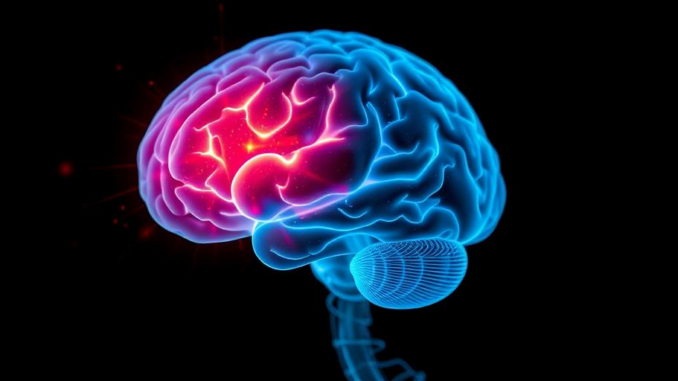
Abstract
Gliomas represent a heterogeneous group of primary brain tumors arising from glial cells, encompassing a wide spectrum of malignancy. While lower-grade gliomas often exhibit indolent growth, high-grade gliomas (HGGs), particularly glioblastoma (GBM), pose a significant clinical challenge due to their aggressive nature, infiltrative growth pattern, and resistance to conventional therapies. This review provides a comprehensive overview of gliomas, encompassing their classification, molecular pathogenesis, clinical presentation, diagnostic modalities, treatment strategies, and prognosis. We delve into the complexities of HGGs, focusing on the challenges posed by the blood-brain barrier (BBB), tumor heterogeneity, and therapeutic resistance. Furthermore, we explore ongoing research efforts aimed at developing novel therapeutic approaches, including targeted therapies, immunotherapies, and gene therapies, with the ultimate goal of improving patient outcomes.
Many thanks to our sponsor Maggie who helped us prepare this research report.
1. Introduction
Gliomas are the most common type of primary brain tumor, accounting for approximately 30% of all brain tumors and 80% of malignant brain tumors [1]. They originate from glial cells, which are the supportive cells of the central nervous system, including astrocytes, oligodendrocytes, and ependymal cells. The World Health Organization (WHO) classifies gliomas based on their histological features and molecular characteristics, grading them from I to IV, with grade I being the least aggressive and grade IV being the most aggressive [2]. The clinical behavior and prognosis of gliomas vary significantly depending on the grade and specific subtype. While lower-grade gliomas (LGGs) may exhibit indolent growth and relatively favorable outcomes, HGGs, especially GBM (WHO grade IV), are characterized by rapid proliferation, diffuse infiltration, and resistance to conventional therapies, leading to poor prognosis.
The diagnosis and management of gliomas require a multidisciplinary approach involving neurosurgeons, neurologists, radiation oncologists, medical oncologists, and neuropathologists. Advances in neuroimaging techniques, molecular diagnostics, and surgical techniques have improved the accuracy of diagnosis and the extent of resection, but the development of effective therapies for HGGs remains a significant challenge. This review aims to provide a comprehensive overview of gliomas, focusing on their classification, pathogenesis, diagnosis, treatment, and ongoing research efforts.
Many thanks to our sponsor Maggie who helped us prepare this research report.
2. Classification of Gliomas
The WHO classification of central nervous system (CNS) tumors has undergone significant revisions in recent years, incorporating molecular parameters in addition to traditional histological features [2, 3]. This integrated approach has refined the diagnostic accuracy and provided a more precise understanding of the biological behavior of gliomas.
2.1 Astrocytic Tumors
Astrocytomas are the most common type of glioma, originating from astrocytes. They are classified into several subtypes based on their histological features and molecular markers:
-
Pilocytic Astrocytoma (WHO Grade I): Typically occurs in children and young adults, often located in the cerebellum or hypothalamus. Characterized by slow growth and favorable prognosis, often curable with surgical resection [4]. Frequent mutations involve the MAPK pathway. These tumors often exhibit a circumscribed border and are amenable to gross total resection, leading to long-term survival in many cases.
-
Diffuse Astrocytoma (WHO Grade II): Infiltrative tumor with slow growth, often located in the cerebral hemispheres. Can progress to higher grades over time. Characterized by IDH mutations in many cases [5]. The treatment strategy usually involves surgical resection followed by observation or adjuvant therapy depending on the extent of resection and molecular features.
-
Anaplastic Astrocytoma (WHO Grade III): More aggressive than diffuse astrocytoma, with increased cellularity, nuclear atypia, and mitotic activity. Also frequently associated with IDH mutations. Adjuvant radiation and chemotherapy are commonly used after surgery to delay progression. The therapeutic landscape is evolving with ongoing clinical trials evaluating novel targeted therapies and immunotherapeutic approaches.
-
Glioblastoma (GBM) (WHO Grade IV): The most aggressive and common type of glioma. Characterized by rapid growth, necrosis, and microvascular proliferation. Can arise de novo (primary GBM) or progress from lower-grade astrocytomas (secondary GBM). Primary GBM is frequently associated with EGFR amplification and PTEN deletion, while secondary GBM often harbors IDH mutations. GBM remains a significant clinical challenge due to its aggressive nature, infiltrative growth pattern, and resistance to conventional therapies [6].
2.2 Oligodendroglial Tumors
Oligodendrogliomas originate from oligodendrocytes and are characterized by their distinct histological features and molecular profile. They are typically located in the cerebral hemispheres and often present with seizures.
-
Oligodendroglioma (WHO Grade II): Characterized by uniform cells with perinuclear halos (“fried-egg” appearance) and branching capillaries. Almost always harbors IDH mutations and 1p/19q codeletion, which is associated with chemosensitivity and improved prognosis [7].
-
Anaplastic Oligodendroglioma (WHO Grade III): More aggressive than oligodendroglioma, with increased cellularity, nuclear atypia, and mitotic activity. Also harbors IDH mutations and 1p/19q codeletion. The optimal treatment strategy involves maximal surgical resection followed by radiation and chemotherapy, often with temozolomide and procarbazine, lomustine, and vincristine (PCV) [8].
2.3 Ependymal Tumors
Ependymomas arise from ependymal cells, which line the ventricles of the brain and the central canal of the spinal cord. They are more common in children than adults and can occur in various locations within the CNS.
-
Ependymoma (WHO Grade II): Characterized by perivascular pseudorosettes and ependymal rosettes. Several molecular subtypes exist, with varying clinical behavior [9].
-
Anaplastic Ependymoma (WHO Grade III): More aggressive than ependymoma, with increased cellularity, nuclear atypia, and mitotic activity. Requires aggressive treatment strategies including surgical resection and radiation therapy.
Many thanks to our sponsor Maggie who helped us prepare this research report.
3. Etiology and Pathogenesis
The exact causes of gliomas are not fully understood, but a combination of genetic and environmental factors is believed to contribute to their development. Genetic predisposition plays a role in some cases, particularly in individuals with inherited cancer syndromes such as neurofibromatosis type 1 (NF1) and Li-Fraumeni syndrome [10]. These syndromes are associated with an increased risk of developing various types of tumors, including gliomas. Germline mutations in genes involved in DNA repair, such as TP53 and BRCA1/2, have also been implicated in glioma development [11].
Environmental factors, such as exposure to ionizing radiation, have been linked to an increased risk of gliomas [12]. However, the association between other environmental factors, such as diet and lifestyle, and glioma risk remains unclear. The role of infectious agents in glioma development is also being investigated.
At the molecular level, gliomas are characterized by a complex interplay of genetic and epigenetic alterations that drive tumor initiation, progression, and resistance to therapy. These alterations affect various signaling pathways involved in cell growth, proliferation, survival, and differentiation. Key signaling pathways implicated in glioma pathogenesis include the receptor tyrosine kinase (RTK)/RAS/MAPK pathway, the PI3K/AKT/mTOR pathway, and the p53 and RB pathways [13].
Mutations in genes encoding RTKs, such as EGFR and PDGFR, are frequently observed in GBM, leading to constitutive activation of downstream signaling pathways [14]. Activation of the PI3K/AKT/mTOR pathway promotes cell survival and proliferation, while inactivation of the p53 and RB pathways disrupts cell cycle control and apoptosis. Furthermore, epigenetic modifications, such as DNA methylation and histone modifications, can alter gene expression patterns and contribute to glioma development [15].
Many thanks to our sponsor Maggie who helped us prepare this research report.
4. Clinical Presentation and Diagnosis
The clinical presentation of gliomas varies depending on the location, size, and growth rate of the tumor. Common symptoms include headaches, seizures, neurological deficits (such as weakness, speech difficulties, and visual disturbances), and cognitive impairment [16]. The onset of symptoms can be gradual or abrupt, depending on the aggressiveness of the tumor. In some cases, gliomas may be asymptomatic and discovered incidentally during imaging studies performed for other reasons.
4.1 Neuroimaging
Neuroimaging plays a crucial role in the diagnosis, staging, and monitoring of gliomas. Magnetic resonance imaging (MRI) is the preferred imaging modality for evaluating brain tumors due to its superior soft tissue resolution and ability to detect subtle changes in brain parenchyma. MRI sequences commonly used in glioma imaging include T1-weighted, T2-weighted, fluid-attenuated inversion recovery (FLAIR), and diffusion-weighted imaging (DWI) [17].
Contrast-enhanced MRI is used to assess the integrity of the BBB and identify areas of tumor enhancement. Gadolinium-based contrast agents are typically used, but concerns about gadolinium deposition in the brain have led to the development of alternative contrast agents. Advanced MRI techniques, such as perfusion-weighted imaging (PWI) and magnetic resonance spectroscopy (MRS), can provide additional information about tumor vascularity and metabolic activity [18].
4.2 Biopsy and Molecular Analysis
Biopsy is essential for obtaining tissue samples for histological examination and molecular analysis. Stereotactic biopsy is a minimally invasive procedure that allows for precise targeting of suspicious areas within the brain [19]. The tissue samples are then examined by a neuropathologist to determine the tumor type, grade, and presence of specific molecular markers. Molecular analysis includes techniques such as immunohistochemistry (IHC), fluorescence in situ hybridization (FISH), and next-generation sequencing (NGS) [20].
NGS allows for comprehensive profiling of the tumor genome, identifying mutations, copy number alterations, and gene fusions. This information can be used to refine the diagnosis, predict prognosis, and guide treatment decisions. The identification of IDH mutations and 1p/19q codeletion is particularly important for the diagnosis and management of oligodendrogliomas. Furthermore, the detection of MGMT promoter methylation status in GBM is crucial for predicting response to temozolomide chemotherapy [21].
Many thanks to our sponsor Maggie who helped us prepare this research report.
5. Treatment Strategies
The treatment of gliomas depends on the tumor type, grade, location, and extent of resection, as well as the patient’s age, overall health, and neurological function. The primary goals of treatment are to maximize tumor control, preserve neurological function, and improve quality of life. A multidisciplinary approach involving neurosurgeons, radiation oncologists, and medical oncologists is essential for optimal management.
5.1 Surgical Resection
Surgical resection is the first-line treatment for most gliomas, with the goal of removing as much tumor as possible while preserving neurological function [22]. The extent of resection is a critical prognostic factor, particularly in HGGs. Gross total resection (GTR), defined as the complete removal of all visible tumor on postoperative imaging, is associated with improved survival outcomes. However, achieving GTR can be challenging due to the infiltrative nature of gliomas and their proximity to critical brain structures.
Intraoperative MRI (iMRI) can be used to guide surgical resection and maximize the extent of tumor removal. iMRI provides real-time imaging during surgery, allowing the surgeon to visualize the tumor margins and identify residual tumor [23]. Awake craniotomy, in which the patient is awake during surgery, allows for real-time monitoring of neurological function and reduces the risk of postoperative deficits. Technological advancements such as neuronavigation and ultrasonic aspiration devices are also used to enhance surgical precision and safety.
5.2 Radiation Therapy
Radiation therapy is a standard treatment for HGGs and some LGGs, used to kill residual tumor cells after surgery and prevent recurrence. External beam radiation therapy (EBRT) is the most common type of radiation therapy used for gliomas. EBRT delivers high-energy X-rays to the tumor site from outside the body [24].
Newer radiation techniques, such as intensity-modulated radiation therapy (IMRT) and stereotactic radiosurgery (SRS), allow for more precise targeting of the tumor while minimizing exposure to surrounding healthy tissue. IMRT uses computer-controlled linear accelerators to deliver radiation beams of varying intensities, shaping the radiation dose to conform to the tumor volume. SRS delivers a single, high dose of radiation to a small, well-defined target, typically using a Gamma Knife or linear accelerator [25].
5.3 Chemotherapy
Chemotherapy is an important component of treatment for HGGs, particularly GBM. Temozolomide (TMZ) is the most commonly used chemotherapy drug for GBM. TMZ is an alkylating agent that damages DNA, leading to cell death [26]. It is typically administered concurrently with radiation therapy, followed by adjuvant TMZ for several months.
The MGMT promoter methylation status is a critical predictor of response to TMZ. MGMT encodes a DNA repair enzyme that removes alkyl groups from DNA, thereby reversing the effects of TMZ. MGMT promoter methylation silences the MGMT gene, leading to decreased MGMT protein expression and increased sensitivity to TMZ [27].
Other chemotherapy drugs used for gliomas include carmustine (BCNU), lomustine (CCNU), and procarbazine. These drugs can be administered intravenously or as a wafer implanted directly into the resection cavity (Gliadel wafer). The choice of chemotherapy regimen depends on the tumor type, grade, molecular profile, and patient’s overall health.
5.4 Targeted Therapy
Targeted therapies are drugs that specifically target molecular abnormalities in cancer cells. Several targeted therapies have been developed for gliomas, but their clinical efficacy has been limited. Bevacizumab, a monoclonal antibody that inhibits vascular endothelial growth factor (VEGF), has been approved for the treatment of recurrent GBM [28]. Bevacizumab reduces tumor angiogenesis and edema, but its impact on overall survival is controversial.
Other targeted therapies under investigation for gliomas include EGFR inhibitors, PDGFR inhibitors, and PI3K/AKT/mTOR inhibitors. These drugs have shown promise in preclinical studies, but their clinical efficacy has been limited by the development of resistance and the complex molecular heterogeneity of gliomas [29].
5.5 Immunotherapy
Immunotherapy is a promising new approach to treating gliomas that harnesses the power of the immune system to fight cancer. Immune checkpoint inhibitors, such as anti-PD-1 and anti-CTLA-4 antibodies, have shown activity in some cancers, but their efficacy in GBM has been limited [30]. The immunosuppressive microenvironment of GBM and the poor penetration of immune cells into the brain are major challenges for immunotherapy.
Other immunotherapeutic strategies under investigation for gliomas include adoptive cell therapy, oncolytic viruses, and cancer vaccines. Adoptive cell therapy involves isolating and expanding immune cells from the patient’s blood or tumor, modifying them to target cancer cells, and then infusing them back into the patient [31]. Oncolytic viruses are genetically engineered viruses that selectively infect and kill cancer cells, while also stimulating an immune response against the tumor [32]. Cancer vaccines aim to stimulate the immune system to recognize and attack cancer cells.
Many thanks to our sponsor Maggie who helped us prepare this research report.
6. Prognosis and Challenges
The prognosis of gliomas varies significantly depending on the tumor type, grade, molecular profile, and treatment response. LGGs generally have a more favorable prognosis than HGGs. The median survival for patients with GBM is approximately 15-18 months with standard treatment, but some patients may live longer with aggressive therapy and favorable molecular characteristics [33].
Several factors contribute to the poor prognosis of HGGs, including the infiltrative nature of the tumor, the presence of cancer stem cells, and the development of resistance to therapy. The BBB also poses a major challenge for drug delivery to the brain. The BBB is a highly selective barrier that protects the brain from harmful substances in the bloodstream but also limits the entry of many therapeutic agents [34].
Tumor recurrence is a common problem in gliomas, even after aggressive treatment. Recurrent tumors are often more resistant to therapy than the primary tumor. Several mechanisms contribute to therapeutic resistance, including genetic and epigenetic alterations, activation of alternative signaling pathways, and changes in the tumor microenvironment [35].
Many thanks to our sponsor Maggie who helped us prepare this research report.
7. Ongoing Research Efforts
Ongoing research efforts are focused on developing novel therapeutic approaches to improve the treatment of gliomas. These efforts include:
-
Developing new drug delivery strategies to overcome the BBB: This includes the use of nanoparticles, liposomes, and focused ultrasound to enhance drug penetration into the brain [36].
-
Identifying new molecular targets for targeted therapy: This involves the use of genomics, proteomics, and metabolomics to identify novel targets that are essential for glioma growth and survival [37].
-
Developing more effective immunotherapeutic strategies: This includes the use of adoptive cell therapy, oncolytic viruses, and cancer vaccines to stimulate a robust and durable immune response against gliomas [38].
-
Developing personalized treatment strategies based on the molecular profile of the tumor: This involves the use of NGS and other molecular profiling techniques to tailor treatment to the individual patient [39].
-
Investigating the role of the tumor microenvironment in glioma progression and resistance: This includes the study of the interactions between glioma cells and other cells in the microenvironment, such as immune cells, endothelial cells, and fibroblasts [40].
Many thanks to our sponsor Maggie who helped us prepare this research report.
8. Conclusion
Gliomas represent a heterogeneous group of brain tumors with varying clinical behavior and prognosis. HGGs, particularly GBM, remain a significant clinical challenge due to their aggressive nature, infiltrative growth pattern, and resistance to conventional therapies. Advances in neuroimaging, molecular diagnostics, and surgical techniques have improved the accuracy of diagnosis and the extent of resection, but the development of effective therapies for HGGs remains a critical unmet need.
Ongoing research efforts are focused on developing novel therapeutic approaches, including targeted therapies, immunotherapies, and gene therapies, with the ultimate goal of improving patient outcomes. A better understanding of the molecular pathogenesis of gliomas, the role of the tumor microenvironment, and the mechanisms of therapeutic resistance is essential for developing more effective therapies. Personalized treatment strategies based on the molecular profile of the tumor are likely to play an increasingly important role in the future management of gliomas.
Many thanks to our sponsor Maggie who helped us prepare this research report.
References
[1] Ostrom, Q. T., et al. “CBTRUS statistical report: primary brain and other central nervous system tumors diagnosed in the United States in 2009–2013.” Neuro-oncology 18.suppl 1 (2016): i1-i75.
[2] Louis, D. N., et al. “The 2016 World Health Organization Classification of Tumors of the Central Nervous System: a summary.” Acta neuropathologica 131.6 (2016): 803-820.
[3] Louis, D. N., et al. “2021 WHO classification of tumors of the central nervous system: a summary.” Neuro-oncology 23.8 (2021): 1231-1251.
[4] Rodriguez, F. J., et al. “Pilomyxoid astrocytoma: a review.” Archives of pathology & laboratory medicine 135.6 (2011): 728-733.
[5] Yan, H., et al. “IDH1 and IDH2 mutations in gliomas.” New England Journal of Medicine 360.8 (2009): 765-773.
[6] Stupp, R., et al. “Radiotherapy plus concomitant and adjuvant temozolomide for glioblastoma.” New England Journal of Medicine 352.10 (2005): 987-996.
[7] Cairncross, J. G., et al. “Specific genetic predictors of chemotherapeutic response and survival in patients with anaplastic oligodendrogliomas.” Journal of the National Cancer Institute 90.19 (1998): 1473-1479.
[8] van den Bent, M. J., et al. “PCV chemotherapy for low-grade glioma: the EORTC experience.” European Journal of Cancer 38.12 (2002): 1557-1561.
[9] Pajtler, K. W., et al. “Molecular classification of ependymal tumors across all CNS compartments, histopathological grades, and age groups.” Cancer cell 27.5 (2015): 728-743.
[10] Gutmann, D. H., et al. “The diagnostic evaluation and management of neurofibromatosis 1.” Nature Reviews Neurology 13.9 (2017): 547-555.
[11] Liau, L. M., et al. “Glioma genetics.” Neurosurgical focus 30.4 (2011): E2.
[12] Preston-Martin, S., et al. “Prior exposure to medical or dental x-rays related to gliomas in young adults.” JNCI: Journal of the National Cancer Institute 88.16 (1996): 1120-1124.
[13] Brennan, C. W., et al. “The somatic genomic landscape of glioblastoma.” Cell 147.3 (2011): 643-657.
[14] Furnari, F. B., et al. “Malignant astrocytic gliomas display frequent epidermal growth factor receptor aberrations, some of which are oncogenically constitutive.” Proceedings of the National Academy of Sciences 100.22 (2003): 13032-13037.
[15] Huse, J. T., and D. B. Holland. “Integrated genomic analysis of glioblastoma.” Cancer cell 20.5 (2011): 571-573.
[16] Taal, W., et al. “Quality of life in patients with high-grade gliomas.” Current opinion in neurology 26.6 (2013): 635.
[17] Law, M., et al. “Gliomas: a comprehensive review of imaging characteristics and pitfalls.” American Journal of Roentgenology 180.5 (2003): 1465-1475.
[18] Pope, W. B., et al. “Multi-institutional prospective trial of perfusion MRI as an early and objective biomarker of response in recurrent glioblastoma: report from the American College of Radiology Imaging Network ACRIN 6677.” Journal of Clinical Oncology 27.19 (2009): 3149.
[19] Hall, W. A. “The safety and efficacy of stereotactic biopsy for intracranial lesions.” Cancer 82.9 (1998): 1749-1755.
[20] Aldape, K., et al. “Glioblastoma molecular pathology.” Acta neuropathologica 130.4 (2015): 479-491.
[21] Hegi, M. E., et al. “MGMT gene silencing and benefit from temozolomide in glioblastoma.” New England Journal of Medicine 352.10 (2005): 997-1004.
[22] Berger, M. S., et al. “The effect of extent of resection on survival in patients with glioblastoma multiforme.” Cancer 74.6 (1994): 1784-1791.
[23] Senft, C., et al. “Intraoperative MRI guidance and extent of resection in glioma surgery: a randomised, controlled trial.” The Lancet Oncology 15.9 (2014): 995-1003.
[24] Gilbert, M. R., et al. “A randomized trial of radiation therapy versus radiation therapy plus concurrent and adjuvant temozolomide for newly diagnosed glioblastoma.” New England Journal of Medicine 352.12 (2005): 1197-1206.
[25] Shaw, E., et al. “Whole brain radiation therapy for the treatment of multiple brain metastases: a systematic review.” Journal of Clinical Oncology 24.8 (2006): 1295.
[26] Friedman, H. S., et al. “Temozolomide and treatment of malignant glioma.” Clinical cancer research 6.7 (2000): 2585-2597.
[27] Wick, W., et al. “MGMT testing: the challenges for biomarker-based glioma treatment.” Nature Reviews Clinical Oncology 11.7 (2014): 432-442.
[28] Friedman, H. S., et al. “Bevacizumab alone or in combination with irinotecan in recurrent glioblastoma.” Journal of Clinical Oncology 27.28 (2009): 4733.
[29] Chakravarti, A., et al. “Erlotinib and temozolomide with radiation for newly diagnosed glioblastoma: results of the Radiation Therapy Oncology Group (RTOG) 0424 trial.” International Journal of Radiation Oncology 78.4 (2010): 970-976.
[30] Lim, M., et al. “Trends in clinical trials for glioblastoma: are we making progress?” Clinical cancer research 24.12 (2018): 2754-2761.
[31] O’Rourke, D. M., et al. “Phase I study of regional gene therapy using replication-deficient adenovirus encoding human interferon-β in recurrent malignant gliomas.” Human gene therapy 10.10 (1999): 1601-1613.
[32] Chiocca, E. A., et al. “A phase Ib study of intratumoral oncolytic adenovirus DNX-2401 in patients with recurrent glioblastoma.” Science translational medicine 10.455 (2018): eaat8539.
[33] Lai, A., et al. “Phase II study of cediranib in recurrent glioblastoma.” Clinical cancer research 14.20 (2008): 6429-6435.
[34] Neuwelt, E. A., et al. “Delivery of therapeutic agents to the brain for treatment of primary and metastatic brain tumors.” Molecular interventions 3.1 (2003): 58-68.
[35] Furnari, F. B., et al. “Maladaptive epigenetic reprogramming in resistance to EGFR inhibition in glioblastoma.” Nature communications 6.1 (2015): 1-13.
[36] Poon, W., et al. “Nanoparticles for brain tumor drug delivery.” Advanced drug delivery reviews 65.15 (2013): 2033-2046.
[37] Verhaak, R. G., et al. “Integrated genomic analysis identifies clinically relevant subtypes of glioblastoma characterized by aberrations in PDGFRA, IDH1, EGFR, and NF1.” Cancer cell 17.1 (2010): 98-110.
[38] Reardon, D. A., et al. “Immunomodulatory antibodies block immune checkpoints and overcome resistance to local brain radiotherapy in preclinical models of glioblastoma.” Clinical cancer research 19.6 (2013): 1695-1704.
[39] Batchelor, T. T., et al. “Improved survival with erlotinib plus temozolomide in recurrent glioblastoma: a phase II study.” Journal of Clinical Oncology 26.11 (2008): 1785.
[40] Charles, N. A., et al. “The tumor microenvironment of glioblastoma.” Glioblastoma 215-245 (2011).
Be the first to comment