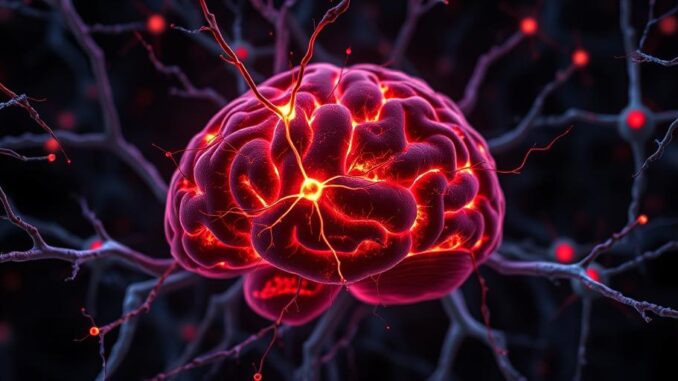
Abstract
Astrocytes, once considered passive support cells in the central nervous system (CNS), are now recognized as active participants in neuronal signaling, synaptic plasticity, and overall brain homeostasis. This research report provides an in-depth examination of astrocyte heterogeneity, covering their distinct subtypes, morphologies, and regional specializations. We will delve into their diverse functions, including neurotransmitter uptake and metabolism, ion homeostasis, gliotransmission, and blood-brain barrier regulation. Furthermore, the report explores the intricate interactions between astrocytes and neurons, highlighting their roles in synapse formation, maturation, and elimination. Critically, we examine the involvement of astrocytes in the pathophysiology of a range of neurological disorders, including neurodegenerative diseases, stroke, traumatic brain injury, and psychiatric conditions. Finally, we discuss potential therapeutic strategies targeting astrocytes for these debilitating conditions.
Many thanks to our sponsor Maggie who helped us prepare this research report.
1. Introduction
The central nervous system (CNS) is a complex network of interconnected cells, where neurons are traditionally considered the primary drivers of information processing. However, glial cells, particularly astrocytes, have emerged as critical players in brain function, challenging the neuron-centric view. Astrocytes, the most abundant glial cell type in the brain, are characterized by their unique morphology, with numerous processes that extend to interact with neurons, synapses, and blood vessels. These intricate interactions position astrocytes as key regulators of the neuronal microenvironment and active participants in neuronal signaling and synaptic plasticity.
Historically, astrocytes were viewed as passive support cells, providing structural support and metabolic sustenance to neurons. However, accumulating evidence over the past few decades has revealed a much more dynamic and multifaceted role for astrocytes. They actively regulate neurotransmitter levels in the synaptic cleft, maintain ion homeostasis, release gliotransmitters that modulate neuronal excitability and synaptic transmission, and contribute to the formation and maintenance of the blood-brain barrier (BBB). Furthermore, astrocytes exhibit remarkable heterogeneity, with distinct subtypes displaying unique morphologies, gene expression profiles, and functional properties. This heterogeneity allows astrocytes to tailor their responses to the specific needs of different brain regions and neuronal circuits.
Dysregulation of astrocyte function has been implicated in a wide range of neurological disorders, including neurodegenerative diseases such as Alzheimer’s disease and Parkinson’s disease, stroke, traumatic brain injury, epilepsy, and psychiatric disorders such as schizophrenia and depression. Understanding the specific roles of astrocytes in these disorders is crucial for developing effective therapeutic strategies. This research report aims to provide a comprehensive overview of astrocyte heterogeneity, function, and dysfunction, highlighting their crucial roles in brain health and disease.
Many thanks to our sponsor Maggie who helped us prepare this research report.
2. Astrocyte Heterogeneity: From Morphology to Molecular Signatures
Astrocytes are not a homogenous population of cells. They exhibit significant heterogeneity in their morphology, gene expression profiles, and functional properties. This heterogeneity is evident across different brain regions, developmental stages, and even within the same region. Understanding astrocyte heterogeneity is essential for deciphering their diverse roles in brain function and disease.
2.1. Regional Heterogeneity
A striking feature of astrocyte heterogeneity is its regional specificity. Astrocytes in different brain regions, such as the cortex, hippocampus, and cerebellum, exhibit distinct morphologies and gene expression patterns. For example, protoplasmic astrocytes, found predominantly in the gray matter, have highly branched processes that ensheath synapses and interact with neurons. In contrast, fibrous astrocytes, located primarily in the white matter, have fewer and less branched processes that are closely associated with myelinated axons.
Moreover, astrocytes in different brain regions express different levels of key astrocyte markers, such as glial fibrillary acidic protein (GFAP) and S100β. GFAP expression, in particular, is highly variable across brain regions and can be upregulated in response to injury or disease. S100β, a calcium-binding protein, is involved in various astrocyte functions, including neuronal survival and synapse formation. The differential expression of these markers reflects the specialized functions of astrocytes in different brain regions.
2.2. Morphological Heterogeneity
The morphology of astrocytes is closely related to their function. The highly branched processes of protoplasmic astrocytes allow them to closely interact with multiple synapses and neurons, enabling them to regulate synaptic transmission and neuronal excitability. The processes of astrocytes also form perivascular endfeet, which are closely associated with blood vessels and contribute to the formation and maintenance of the blood-brain barrier (BBB). Fibrous astrocytes, with their close association with myelinated axons, are thought to play a role in axonal support and myelination.
Recent studies have also identified specialized subtypes of astrocytes with unique morphologies. For example, interlaminar astrocytes in the superficial layers of the cerebral cortex have long, vertically oriented processes that span multiple cortical layers. These astrocytes are thought to play a role in integrating information across different cortical layers.
2.3. Molecular Heterogeneity
Advances in single-cell RNA sequencing (scRNA-seq) have revealed unprecedented insights into the molecular heterogeneity of astrocytes. These studies have identified distinct astrocyte subtypes based on their unique gene expression profiles. For example, scRNA-seq studies have identified subpopulations of astrocytes that are enriched for genes involved in specific functions, such as glutamate metabolism, potassium buffering, or inflammatory responses.
Furthermore, astrocyte heterogeneity is not static but can be dynamically regulated in response to changes in the neuronal microenvironment. For example, astrocytes can undergo reactive astrogliosis in response to injury or disease, leading to changes in their gene expression profiles and morphology. Reactive astrocytes can exhibit both beneficial and detrimental effects, depending on the context and the specific subtypes of astrocytes involved.
2.4. Functional Implications of Heterogeneity
The heterogeneity of astrocytes has profound implications for their functional roles in the brain. Different astrocyte subtypes may perform distinct functions, contributing to the overall complexity of astrocyte-neuron interactions and brain function. For example, astrocytes that are enriched for glutamate transporters may be particularly important for regulating glutamate levels in the synaptic cleft, while astrocytes that express high levels of potassium channels may be crucial for maintaining potassium homeostasis. The diverse functions of different astrocyte subtypes are essential for maintaining neuronal health, regulating synaptic transmission, and coordinating brain activity.
Many thanks to our sponsor Maggie who helped us prepare this research report.
3. Astrocyte Functions: Guardians of the Neuronal Microenvironment
Astrocytes perform a wide range of functions that are essential for maintaining the health and function of neurons and the overall integrity of the brain. These functions include neurotransmitter uptake and metabolism, ion homeostasis, gliotransmission, and blood-brain barrier regulation.
3.1. Neurotransmitter Uptake and Metabolism
Astrocytes play a critical role in regulating neurotransmitter levels in the synaptic cleft. They express a variety of neurotransmitter transporters, including glutamate transporters (GLT-1 and GLAST), GABA transporters (GAT-1 and GAT-3), and adenosine transporters. These transporters remove neurotransmitters from the synaptic cleft, preventing excessive neuronal excitation or inhibition and ensuring proper synaptic transmission.
Glutamate, the major excitatory neurotransmitter in the brain, is particularly important for astrocyte regulation. Astrocytes express high levels of GLT-1 and GLAST, which rapidly remove glutamate from the synaptic cleft. Glutamate is then converted to glutamine by glutamine synthetase, an enzyme that is exclusively expressed in astrocytes. Glutamine is then transported back to neurons, where it is converted back to glutamate, completing the glutamate-glutamine cycle. This cycle is essential for maintaining glutamate homeostasis and preventing excitotoxicity, a condition in which excessive glutamate levels lead to neuronal damage or death.
Astrocytes also metabolize other neurotransmitters, such as GABA and adenosine. GABA is metabolized by GABA transaminase, while adenosine is metabolized by adenosine deaminase. These metabolic pathways contribute to the regulation of neurotransmitter levels and the overall balance of excitation and inhibition in the brain.
3.2. Ion Homeostasis
Astrocytes play a crucial role in maintaining ion homeostasis in the neuronal microenvironment. They express a variety of ion channels and transporters that regulate the concentrations of key ions, such as potassium (K+), sodium (Na+), and calcium (Ca2+). Potassium is particularly important for astrocyte regulation, as changes in extracellular potassium levels can significantly affect neuronal excitability. Astrocytes express a variety of potassium channels, including Kir4.1, which is highly permeable to potassium. These channels allow astrocytes to rapidly buffer changes in extracellular potassium levels, preventing neuronal hyperexcitability and maintaining stable neuronal firing patterns.
Astrocytes also regulate calcium homeostasis. They express a variety of calcium channels and intracellular calcium stores that allow them to respond to neuronal activity and release calcium-dependent gliotransmitters. Calcium signaling in astrocytes is complex and can involve both localized calcium microdomains and global calcium waves that propagate throughout the astrocyte network.
3.3. Gliotransmission
Astrocytes communicate with neurons and other glial cells through the release of gliotransmitters, signaling molecules that modulate neuronal excitability and synaptic transmission. Gliotransmitters include glutamate, ATP, D-serine, and GABA. Astrocytes release gliotransmitters in response to neuronal activity or other stimuli, such as changes in calcium levels. Glutamate released from astrocytes can activate neuronal glutamate receptors, modulating neuronal excitability and synaptic plasticity. ATP released from astrocytes can activate purinergic receptors on neurons and other glial cells, triggering a variety of downstream signaling cascades. D-serine released from astrocytes is a co-agonist of the NMDA receptor, a glutamate receptor that is critical for synaptic plasticity and learning and memory. GABA released from astrocytes can activate neuronal GABA receptors, inhibiting neuronal excitability.
The mechanisms by which astrocytes release gliotransmitters are complex and can involve both vesicular and non-vesicular pathways. Vesicular release involves the packaging of gliotransmitters into vesicles, which then fuse with the plasma membrane to release their contents. Non-vesicular release can occur through a variety of mechanisms, including diffusion through channels or transporters and release through membrane pores.
3.4. Blood-Brain Barrier Regulation
Astrocytes play a crucial role in forming and maintaining the blood-brain barrier (BBB), a highly selective barrier that protects the brain from harmful substances in the blood. Astrocyte endfeet surround blood vessels and contribute to the tight junctions between endothelial cells, which are the main components of the BBB. Astrocytes also regulate the expression of transporters and enzymes in endothelial cells that control the passage of molecules across the BBB. Astrocytes release factors, such as vascular endothelial growth factor (VEGF), that promote angiogenesis and BBB formation. Dysregulation of astrocyte function can lead to BBB breakdown, which can contribute to neuronal damage and inflammation.
Many thanks to our sponsor Maggie who helped us prepare this research report.
4. Astrocyte-Neuron Interactions: A Dynamic Partnership
Astrocytes and neurons engage in a dynamic partnership, with astrocytes actively modulating neuronal activity and synaptic transmission. These interactions are critical for synapse formation, maturation, and elimination, as well as for the regulation of neuronal excitability and synaptic plasticity.
4.1. Synapse Formation and Maturation
Astrocytes play a critical role in synapse formation and maturation during development. They secrete factors, such as thrombospondins and glypicans, that promote synapse formation and stabilize synaptic connections. Astrocytes also regulate the expression of synaptic proteins in neurons, ensuring proper synaptic function. Furthermore, astrocytes contribute to the maturation of synapses by promoting the formation of dendritic spines, the postsynaptic structures that receive synaptic inputs.
4.2. Synapse Elimination
Astrocytes also participate in synapse elimination, a process that is essential for refining neuronal circuits during development. Astrocytes engulf and eliminate synapses through phagocytosis, a process that is regulated by complement factors and other signaling molecules. Synapse elimination is important for removing weak or inappropriate synapses and strengthening strong, relevant connections.
4.3. Regulation of Neuronal Excitability
Astrocytes regulate neuronal excitability through a variety of mechanisms, including neurotransmitter uptake, ion homeostasis, and gliotransmission. By regulating neurotransmitter levels in the synaptic cleft, astrocytes can control the strength and duration of synaptic transmission. By maintaining ion homeostasis, astrocytes can prevent neuronal hyperexcitability or hyperpolarization. By releasing gliotransmitters, astrocytes can directly modulate neuronal excitability and synaptic plasticity.
4.4. Modulation of Synaptic Plasticity
Astrocytes play a crucial role in synaptic plasticity, the ability of synapses to strengthen or weaken over time. Synaptic plasticity is the cellular basis of learning and memory. Astrocytes modulate synaptic plasticity through a variety of mechanisms, including the release of gliotransmitters, the regulation of calcium signaling in neurons, and the modulation of gene expression in neurons. For example, D-serine released from astrocytes is a co-agonist of the NMDA receptor, a glutamate receptor that is critical for long-term potentiation (LTP), a form of synaptic plasticity that is thought to underlie learning and memory.
Many thanks to our sponsor Maggie who helped us prepare this research report.
5. Astrocyte Dysfunction in Neurological Disorders
Dysregulation of astrocyte function has been implicated in a wide range of neurological disorders, including neurodegenerative diseases, stroke, traumatic brain injury, epilepsy, and psychiatric disorders. Understanding the specific roles of astrocytes in these disorders is crucial for developing effective therapeutic strategies.
5.1. Neurodegenerative Diseases
Astrocytes play a complex and often dual role in neurodegenerative diseases such as Alzheimer’s disease, Parkinson’s disease, and Huntington’s disease. In the early stages of these diseases, astrocytes can exhibit neuroprotective effects, promoting neuronal survival and clearing toxic protein aggregates. However, as the disease progresses, astrocytes can become reactive and contribute to neuroinflammation and neuronal damage. In Alzheimer’s disease, astrocytes accumulate around amyloid plaques and release inflammatory cytokines that contribute to neuronal dysfunction and cognitive decline. In Parkinson’s disease, astrocytes contribute to the loss of dopaminergic neurons by releasing inflammatory mediators and disrupting dopamine signaling. In Huntington’s disease, astrocytes contribute to neuronal dysfunction and cell death by releasing excitotoxic substances and disrupting glutamate homeostasis.
5.2. Stroke
Following a stroke, astrocytes undergo reactive astrogliosis and contribute to both neuroprotection and neurotoxicity. In the acute phase of stroke, astrocytes can protect neurons by scavenging glutamate and maintaining ion homeostasis. However, in the chronic phase of stroke, reactive astrocytes can contribute to scar formation and inhibit axonal regeneration. Furthermore, astrocytes can release inflammatory cytokines that exacerbate neuronal damage and inflammation.
5.3. Traumatic Brain Injury
Traumatic brain injury (TBI) can lead to widespread astrocyte damage and dysfunction. Astrocytes contribute to both the acute and chronic consequences of TBI. In the acute phase of TBI, astrocytes can release inflammatory mediators and contribute to edema formation. In the chronic phase of TBI, astrocytes can contribute to scar formation and inhibit axonal regeneration. Furthermore, astrocytes can disrupt the BBB, leading to increased permeability and inflammation.
5.4. Epilepsy
Astrocytes play a crucial role in regulating neuronal excitability and preventing seizures. Dysregulation of astrocyte function has been implicated in the pathogenesis of epilepsy. For example, mutations in genes that encode astrocyte potassium channels can lead to decreased potassium buffering capacity, increasing neuronal excitability and promoting seizures. Furthermore, astrocytes can release gliotransmitters that modulate neuronal excitability and contribute to seizure generation. Reactive astrocytes can also contribute to the formation of glial scars, which can disrupt neuronal circuits and promote seizures.
5.5. Psychiatric Disorders
Mounting evidence suggests that astrocytes play a role in the pathophysiology of psychiatric disorders such as schizophrenia, depression, and autism spectrum disorder (ASD). In schizophrenia, astrocytes exhibit altered gene expression and morphology, and they may contribute to the disrupted neuronal circuits that underlie the symptoms of the disorder. In depression, astrocytes can release inflammatory cytokines and disrupt glutamate signaling, contributing to neuronal dysfunction and mood dysregulation. In ASD, astrocytes exhibit altered development and function, and they may contribute to the social and cognitive deficits that characterize the disorder.
Many thanks to our sponsor Maggie who helped us prepare this research report.
6. Therapeutic Strategies Targeting Astrocytes
Given the crucial roles of astrocytes in brain function and disease, targeting astrocytes represents a promising therapeutic strategy for a wide range of neurological disorders. Several therapeutic approaches are being investigated, including:
6.1. Modulating Astrocyte Reactivity
Controlling astrocyte reactivity is a key therapeutic goal. This can involve inhibiting the activation of pro-inflammatory astrocytes and promoting the activation of neuroprotective astrocytes. Several drugs are being developed to modulate astrocyte reactivity, including anti-inflammatory agents, antioxidants, and growth factors.
6.2. Enhancing Astrocyte Function
Enhancing astrocyte function can improve neuronal health and resilience. This can involve promoting neurotransmitter uptake, improving ion homeostasis, and increasing the release of neurotrophic factors. Several drugs are being developed to enhance astrocyte function, including glutamate transporter activators, potassium channel modulators, and growth factor agonists.
6.3. Gene Therapy
Gene therapy can be used to deliver therapeutic genes to astrocytes, correcting genetic defects or enhancing specific astrocyte functions. This approach holds promise for treating genetic disorders that affect astrocyte function, as well as for delivering neuroprotective genes to astrocytes in neurodegenerative diseases.
6.4. Cell Transplantation
Cell transplantation involves transplanting healthy astrocytes into the brain to replace damaged or dysfunctional astrocytes. This approach has shown promise in preclinical studies for treating stroke, TBI, and neurodegenerative diseases.
6.5. Targeting Gliotransmission
Modulating gliotransmission can be used to control neuronal excitability and synaptic plasticity. This can involve inhibiting the release of pro-excitatory gliotransmitters, such as glutamate, or promoting the release of neuroprotective gliotransmitters, such as D-serine. Several drugs are being developed to target gliotransmission, including glutamate receptor antagonists and D-serine agonists.
Many thanks to our sponsor Maggie who helped us prepare this research report.
7. Conclusion
Astrocytes are emerging as key players in brain function and disease. Their diverse functions, including neurotransmitter uptake, ion homeostasis, gliotransmission, and BBB regulation, are essential for maintaining neuronal health and regulating synaptic transmission. Dysregulation of astrocyte function has been implicated in a wide range of neurological disorders. Understanding the specific roles of astrocytes in these disorders is crucial for developing effective therapeutic strategies. Targeting astrocytes represents a promising therapeutic approach for a wide range of neurological disorders.
Many thanks to our sponsor Maggie who helped us prepare this research report.
References
- Barres, B. A. (2008). The mystery and magic of glia: a perspective on their roles in health and disease. Neuron, 60(3), 430-440.
- Khakh, B. S., & McCarthy, K. D. (2015). Astrocyte calcium signaling: from observations to functions. Cold Spring Harbor Perspectives in Biology, 7(5), a020409.
- Sofroniew, M. V. (2009). Molecular dissection of reactive astrogliosis and glial scar formation. Trends in Neurosciences, 32(12), 638-647.
- Clarke, L. E., & Barres, B. A. (2013). Emerging roles of astrocytes in neural circuit development. Nature Reviews Neuroscience, 14(5), 311-321.
- Chung, W. S., Allen, N. J., & Eroglu, C. (2015). Astrocytes control synapse formation, function, and elimination. Cold Spring Harbor Perspectives in Biology, 7(9), a020375.
- Allen, N. J., & Lyons, D. A. (2018). Gliogenesis and the formation of functional astrocyte networks. Current Opinion in Neurobiology, 50, 72-80.
- Escartin, C., Galea, E., Lakatos, A., O’Callaghan, J. P., Petzold, G. C., Serrano-Pozo, A., … & Volterra, A. (2021). Reactive astrocyte nomenclature, definitions, and future directions. Nature Neuroscience, 24(3), 312-325.
- Verkhratsky, A., Nedergaard, M., & Hertz, L. (2015). Why are astrocytes important?. ASN Neuro, 7(6), 1759091415623854.
- Liddelow, S. A., Guttenplan, C. A., Clarke, L. E., Bennett, F. C., Bohlen, C. J., Schirmer, L., … & Barres, B. A. (2017). Neurotoxic reactive astrocytes are induced by microglia and promote neuronal death. Nature, 541(7638), 481-487.
- Clarke, L. E., Liddelow, S. A., Chakraborty, C., Münch, A. E., Heiman, M., Barres, B. A. (2018). Normal aging induces A1-like astrocyte reactivity that is distinct from that seen in Alzheimer’s disease. Proceedings of the National Academy of Sciences, 115(4), E1896-E1905.
- Ben Haim, L., Rowitch, D. H., & Kriegstein, A. R. (2015). Astrocyte development and function in the human brain. Glia, 63(8), 1231-1243.
- Khakh, B. S. (2019). Molecular and cellular logic of glutamate escape from astrocytes. Neuron, 104(3), 438-454.
- Santello, M., & Volterra, A. (2009). Gliotransmission at the tripartite synapse. Glia, 57(6), 511-525.
- Sofroniew, M. V., & Vinters, H. V. (2010). Astrocytes: biology and pathology. Acta Neuropathologica, 119(1), 7-35.
- Verkhratsky, A., & Butt, A. M. (2013). Glia. John Wiley & Sons.
- Chung, W. S., Verghese, P. B., Chakraborty, C., Joung, J., Hyman, B. T., Ulrich, J. D., & Holtzman, D. M. (2016). Novel allele-dependent role for APOE in controlling the rate of synapse pruning by astrocytes. Proceedings of the National Academy of Sciences, 113(37), 10186-10191.
- Guttenplan, C. A., Weigel, M. K., Adler, D. I., Crunkhorn, S., Patel, M. K., Taga, M. F., … & Khakh, B. S. (2021). Transcriptomic profiling reveals striking and unexpected diversity of mouse cortical astrocytes. Nature Neuroscience, 24(3), 355-368.
- Allen, N. J., Tsai, L. H., & Barres, B. A. (2002). Thrombospondins are astrocyte-secreted proteins that promote synaptogenesis. Cell, 111(2), 275-287.
- Lobsiger, C. S., & Cleveland, D. W. (2007). Glial cells as intrinsic components of non-cell-autonomous neurodegenerative disease. Nature Neuroscience, 10(11), 1355-1360.
- Pekny, M., & Nilsson, M. (2005). Astrocyte activation and reactive gliosis: too good to be bad?. Glia, 50(4), 427-434.
- Seifert, G., Carmignoto, G., & Steinhäuser, C. (2009). Astrocyte dysfunction in epilepsy. Brain Research Reviews, 63(1-2), 196-211.
- Hertz, L., & Holmhøj, J. E. (2006). Role of astrocytes in glutamate homeostasis in the brain. Neurochemistry International, 48(6-7), 443-458.
Be the first to comment