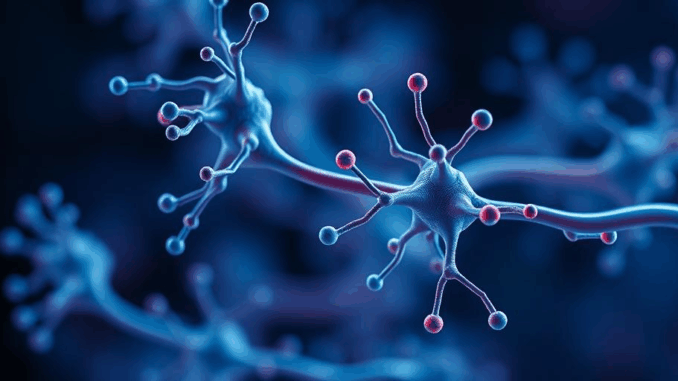
Abstract
Agonists are a cornerstone of modern pharmacology, acting as key-in-lock molecules that bind to receptors and initiate cellular signaling pathways. This report provides a comprehensive overview of agonist pharmacology, encompassing the fundamental mechanisms of action, diverse therapeutic applications, and emerging research directions. We delve into the complexities of receptor-agonist interactions, including affinity, efficacy, and the nuanced concept of biased agonism. Furthermore, we explore the impact of agonists across various organ systems, highlighting examples from established therapies such as beta-adrenergic agonists for asthma to cutting-edge applications like GLP-1 receptor agonists for metabolic disorders and potential novel uses in addiction treatment. The report critically examines the current landscape of agonist development, addressing challenges related to selectivity, bioavailability, and the mitigation of adverse effects. Finally, we explore future research avenues, including the rational design of novel agonists, the exploitation of allosteric modulation, and the application of systems pharmacology to optimize agonist-based therapies. This review aims to provide experts in the field with an up-to-date and in-depth understanding of agonist pharmacology, fostering innovation and paving the way for the development of more effective and targeted therapeutics.
Many thanks to our sponsor Maggie who helped us prepare this research report.
1. Introduction
The interaction between a drug and its target, most commonly a receptor, is the fundamental basis of pharmacology. Among the various classes of drugs, agonists hold a prominent position. An agonist, by definition, is a molecule that binds to a receptor and activates it, triggering a biological response. This seemingly simple interaction underpins a vast array of therapeutic interventions, from managing chronic conditions like hypertension and diabetes to treating acute illnesses like infections and pain. While the concept of agonism has been well-established for decades, the understanding of its intricacies has evolved significantly. Initial models focused on a binary view of receptor activation – either “on” or “off.” However, contemporary research has revealed a more complex picture, highlighting the importance of receptor conformations, signaling bias, and the influence of the cellular environment. This complexity offers opportunities to refine drug design and develop agonists with improved efficacy, selectivity, and safety profiles.
This report provides a comprehensive review of agonist pharmacology, covering the fundamental principles, therapeutic applications, challenges, and future directions. We will begin by discussing the molecular mechanisms of agonist-receptor interactions, including the concepts of affinity, efficacy, and biased agonism. Then, we will explore the therapeutic applications of agonists across various organ systems, with particular emphasis on the GLP-1 agonists as a potential treatment for addiction and metabolic disorders, and other relevant examples. We will also address the challenges associated with agonist development, such as off-target effects, bioavailability, and the emergence of drug resistance. Finally, we will look towards the future of agonist research, highlighting emerging strategies for the rational design of novel agonists and the application of systems pharmacology to optimize agonist-based therapies. Our goal is to provide a current and thorough overview of agonist pharmacology to professionals, encouraging innovation and promoting the creation of more focused and efficient therapies.
Many thanks to our sponsor Maggie who helped us prepare this research report.
2. Molecular Mechanisms of Agonist Action
The action of an agonist hinges on its ability to bind to a receptor and induce a conformational change that activates downstream signaling pathways. This interaction is governed by several key factors, including affinity, efficacy, and selectivity.
2.1 Affinity and Receptor Binding
Affinity refers to the strength of the interaction between an agonist and its receptor. It is typically quantified by the dissociation constant (Kd), which represents the concentration of agonist required to occupy 50% of the receptor population at equilibrium. A lower Kd value indicates a higher affinity. The binding of an agonist to a receptor is influenced by various forces, including hydrogen bonds, van der Waals interactions, and electrostatic interactions. The specific amino acid residues within the receptor’s binding pocket play a crucial role in determining the affinity of an agonist. High-affinity agonists exhibit a prolonged residence time on the receptor, leading to sustained activation of downstream signaling pathways.
2.2 Efficacy and Signal Transduction
Efficacy, sometimes referred to as intrinsic activity, describes the ability of an agonist to activate a receptor and elicit a maximal biological response. In essence, it is the degree to which an agonist triggers a change in receptor conformation to produce a downstream effect. Agonists can be classified based on their efficacy: full agonists produce the maximal possible response, partial agonists produce a submaximal response even at saturating concentrations, and inverse agonists produce an effect opposite to that of a full agonist (i.e., they reduce basal receptor activity). The efficacy of an agonist is dependent on the specific receptor subtype, the cellular context, and the downstream signaling pathways involved.
2.3 Biased Agonism
Traditional models of receptor activation assumed that agonists induce a single, uniform conformational change, leading to the activation of all downstream signaling pathways equally. However, recent research has revealed that agonists can induce different receptor conformations, leading to the preferential activation of specific signaling pathways. This phenomenon is known as biased agonism, or functional selectivity. Biased agonists can selectively activate beneficial signaling pathways while avoiding pathways associated with adverse effects, offering the potential for improved therapeutic outcomes. For example, some opioids are designed to preferentially activate the G-protein-mediated pathway linked to analgesia while avoiding the beta-arrestin pathway associated with respiratory depression. Biased agonism has revolutionized drug discovery, providing a new paradigm for designing drugs with improved efficacy and safety profiles. Rational design of such compounds is very challenging though, and requires advanced structural information and in vitro screening methods.
Many thanks to our sponsor Maggie who helped us prepare this research report.
3. Therapeutic Applications of Agonists
Agonists are widely used in medicine to treat a variety of diseases. Their ability to selectively activate specific receptors makes them valuable therapeutic agents. Here are some notable examples of how agonists are utilized in medical treatments:
3.1 Beta-Adrenergic Agonists for Asthma
Beta-adrenergic agonists, such as salbutamol and formoterol, are commonly used to treat asthma and chronic obstructive pulmonary disease (COPD). These agonists bind to beta2-adrenergic receptors in the smooth muscle of the airways, causing bronchodilation and relieving symptoms of wheezing and shortness of breath. Short-acting beta-agonists (SABAs) like salbutamol provide quick relief during acute asthma attacks, while long-acting beta-agonists (LABAs) like formoterol provide sustained bronchodilation and are used for long-term asthma control in combination with inhaled corticosteroids.
3.2 Opioid Agonists for Pain Management
Opioid agonists, such as morphine and fentanyl, are potent analgesics used to treat severe pain. They bind to opioid receptors in the central nervous system, reducing pain transmission and perception. However, opioid agonists are also associated with a high risk of addiction and respiratory depression, limiting their long-term use. Research efforts are focused on developing biased opioid agonists that selectively activate the analgesic pathway while minimizing the risk of adverse effects.
3.3 Dopamine Agonists for Parkinson’s Disease
Parkinson’s disease is characterized by the loss of dopamine-producing neurons in the brain. Dopamine agonists, such as pramipexole and ropinirole, are used to treat Parkinson’s disease by directly stimulating dopamine receptors in the brain, compensating for the loss of endogenous dopamine. These agonists can improve motor function and reduce symptoms such as tremors, rigidity, and bradykinesia.
3.4 GLP-1 Receptor Agonists for Metabolic Disorders and Addiction
Glucagon-like peptide-1 (GLP-1) receptor agonists, such as exenatide, liraglutide, semaglutide and tirzepatide, are increasingly recognized for their therapeutic potential in both metabolic disorders and potentially addiction. Initially developed for treating type 2 diabetes, these agonists stimulate the GLP-1 receptor, which is expressed in the pancreas, brain, and gastrointestinal tract. In diabetes, they enhance insulin secretion, suppress glucagon release, and slow gastric emptying, leading to improved glycemic control. Recent studies have also demonstrated the efficacy of GLP-1 receptor agonists in promoting weight loss. This effect is thought to be mediated by the GLP-1 receptor’s influence on appetite regulation and satiety in the brain.
Intriguingly, emerging preclinical and clinical evidence suggests that GLP-1 receptor agonists may also have a role in treating addiction. The GLP-1 receptor is expressed in brain regions involved in reward and motivation, such as the ventral tegmental area (VTA) and nucleus accumbens (NAc). Studies in animal models have shown that GLP-1 receptor agonists can reduce drug-seeking behavior and relapse in response to cues associated with drugs of abuse. For instance, exenatide and liraglutide have been shown to decrease alcohol consumption and craving in rodents. While the precise mechanisms underlying these effects are still being investigated, it is hypothesized that GLP-1 receptor activation modulates dopamine release in the reward pathway, thereby reducing the rewarding effects of drugs. Clinical trials are underway to evaluate the efficacy of GLP-1 receptor agonists in treating various forms of addiction, including alcohol use disorder, opioid use disorder, and smoking cessation.
The potential use of GLP-1 agonists for addiction treatment is intriguing, offering a novel approach to targeting the underlying neurobiological mechanisms of substance use disorders. It’s worth noting that some researchers argue GLP-1 agonists can transfer the addiction to the medication in the form of a behavioral addiction linked to the weight loss and dopamine release. However, further research is needed to fully elucidate the mechanisms of action, identify optimal treatment strategies, and assess the long-term safety and efficacy of GLP-1 receptor agonists in this context. The development of selective GLP-1 receptor agonists with improved brain penetration could enhance their therapeutic potential for addiction treatment. Further, the study of combination therapies may improve the efficacy of GLP-1 agonists in addiction treatment. The existing clinical trials targeting this treatment are also of interest, as well as the need for larger clinical trials with diverse populations.
3.5 Selective Estrogen Receptor Modulators (SERMs) for Breast Cancer and Osteoporosis
Selective estrogen receptor modulators (SERMs), such as tamoxifen and raloxifene, are agonists that selectively bind to estrogen receptors in different tissues. In breast tissue, tamoxifen acts as an estrogen receptor antagonist, blocking the growth-promoting effects of estrogen and reducing the risk of breast cancer recurrence. In bone tissue, raloxifene acts as an estrogen receptor agonist, promoting bone density and reducing the risk of osteoporosis. SERMs offer a tissue-selective approach to estrogen receptor modulation, minimizing the adverse effects associated with traditional estrogen replacement therapy.
Many thanks to our sponsor Maggie who helped us prepare this research report.
4. Challenges in Agonist Development
While agonists have proven to be valuable therapeutic agents, their development is not without challenges. Here are some of the key hurdles in agonist drug discovery:
4.1 Selectivity and Off-Target Effects
Selectivity is a crucial factor in agonist drug development. An ideal agonist should selectively bind to its intended target receptor while minimizing interactions with other receptors or off-target proteins. Non-selective agonists can cause a variety of adverse effects due to their interactions with unintended targets. For example, first-generation antihistamines, which are non-selective histamine receptor antagonists, can cause drowsiness and cognitive impairment due to their blockade of histamine receptors in the brain. Improving agonist selectivity requires a deep understanding of receptor structure and function, as well as the use of sophisticated screening techniques to identify compounds with the desired selectivity profile. Molecular docking and in silico modeling play a crucial role in predicting the binding affinity and selectivity of agonist candidates.
4.2 Bioavailability and Drug Delivery
Bioavailability refers to the fraction of an administered drug that reaches the systemic circulation in an unchanged form. Poor bioavailability can limit the efficacy of an agonist, even if it has high affinity and efficacy for its target receptor. Factors that can affect bioavailability include poor absorption, first-pass metabolism, and rapid clearance from the body. Various strategies can be used to improve the bioavailability of agonists, including optimizing the formulation, using prodrugs, and employing drug delivery systems such as nanoparticles or liposomes. For example, the bioavailability of some peptide agonists can be improved by conjugating them to polyethylene glycol (PEG), which reduces their degradation and increases their circulation time.
4.3 Tolerance and Drug Resistance
Prolonged exposure to agonists can lead to tolerance, a phenomenon in which the response to the agonist decreases over time. Tolerance can occur due to receptor desensitization, downregulation of receptors, or changes in downstream signaling pathways. Drug resistance, a related phenomenon, can occur when cells become less sensitive to the effects of an agonist. Drug resistance can arise through various mechanisms, including mutations in the receptor gene, increased expression of drug efflux pumps, or activation of alternative signaling pathways. Overcoming tolerance and drug resistance requires a multifaceted approach, including the development of novel agonists with different mechanisms of action, the use of drug combinations, and the implementation of strategies to prevent receptor desensitization.
4.4 Development and Clinical Trials
Agonist drug discovery involves rigorous preclinical and clinical testing to ensure safety and efficacy. Preclinical studies typically involve in vitro and in vivo experiments to assess the agonist’s activity, selectivity, and toxicity. Clinical trials are conducted in humans to evaluate the agonist’s safety, efficacy, and pharmacokinetic properties. Clinical trials are typically divided into three phases: Phase 1 trials assess safety and tolerability in healthy volunteers; Phase 2 trials evaluate efficacy and dose-response in patients with the target disease; and Phase 3 trials confirm efficacy and monitor adverse effects in a larger patient population. The development of new agonists is a long and expensive process, and many drug candidates fail to make it through clinical trials. Therefore, it is essential to have a rigorous drug development strategy and to carefully monitor the safety and efficacy of agonist candidates at each stage of the process.
Many thanks to our sponsor Maggie who helped us prepare this research report.
5. Future Directions in Agonist Research
The field of agonist pharmacology is constantly evolving, with new research and technological advancements paving the way for the development of more effective and targeted therapeutics. Here are some of the exciting future directions in agonist research:
5.1 Rational Design of Novel Agonists
Rational drug design involves using structural information about the target receptor to design agonists with improved affinity, selectivity, and efficacy. Advances in structural biology, such as X-ray crystallography and cryo-electron microscopy, have provided detailed three-dimensional structures of many receptors, enabling researchers to design agonists that precisely fit into the receptor’s binding pocket. Molecular modeling and computational chemistry techniques can be used to predict the binding affinity and efficacy of agonist candidates, guiding the design process. Rational drug design holds the promise of developing agonists with optimized therapeutic properties.
5.2 Exploitation of Allosteric Modulation
Allosteric modulators are molecules that bind to a receptor at a site distinct from the agonist binding site and modulate the receptor’s response to agonists. Positive allosteric modulators (PAMs) enhance the receptor’s response to agonists, while negative allosteric modulators (NAMs) inhibit the receptor’s response. Allosteric modulation offers several advantages over traditional agonist-based therapies. Allosteric modulators can fine-tune receptor activity without directly activating the receptor, reducing the risk of overstimulation or desensitization. Allosteric modulators can also exhibit tissue selectivity, modulating receptor activity only in specific tissues or cell types. The development of allosteric modulators is a promising area of research with the potential to develop more targeted and effective therapeutics.
5.3 Systems Pharmacology Approaches
Systems pharmacology is an emerging field that combines pharmacology, genomics, and systems biology to understand the complex interactions between drugs, receptors, and biological systems. Systems pharmacology approaches can be used to identify novel drug targets, predict drug efficacy and toxicity, and optimize drug dosing regimens. By integrating data from multiple sources, such as genomics, proteomics, and metabolomics, systems pharmacology can provide a holistic view of drug action and guide the development of more effective and personalized therapies. Machine learning and artificial intelligence are increasingly being used in systems pharmacology to analyze large datasets and identify patterns that can predict drug response.
5.4 Nanotechnology and Targeted Drug Delivery
Nanotechnology offers the potential to improve the delivery of agonists to specific tissues or cells. Nanoparticles can be engineered to encapsulate agonists and protect them from degradation, improve their bioavailability, and target them to specific receptors or cell types. For example, nanoparticles can be coated with antibodies or ligands that bind to specific receptors on cancer cells, delivering agonists directly to the tumor. Targeted drug delivery can improve the efficacy of agonists while minimizing their off-target effects, reducing the risk of adverse events. Nanotechnology is rapidly advancing, and it holds great promise for the development of more effective and targeted agonist-based therapies.
5.5 Personalized Medicine
Personalized medicine, also known as precision medicine, aims to tailor treatment to the individual characteristics of each patient. This approach takes into account factors such as genetics, lifestyle, and environmental exposures to predict how a patient will respond to a particular drug. Genetic variations in receptor genes can influence the affinity, efficacy, and signaling bias of agonists. By identifying these genetic variations, clinicians can select the most appropriate agonist for each patient and optimize the dosing regimen. Personalized medicine holds the promise of improving treatment outcomes and reducing the risk of adverse events.
Many thanks to our sponsor Maggie who helped us prepare this research report.
6. Conclusion
Agonists are indispensable tools in pharmacology, serving as the foundation for numerous therapeutic interventions. Their ability to selectively activate receptors and initiate cellular signaling pathways makes them valuable agents for treating a wide range of diseases. However, the development of new agonists is a complex and challenging process, requiring a deep understanding of receptor structure, function, and signaling mechanisms. Overcoming challenges such as selectivity, bioavailability, tolerance, and drug resistance is crucial for developing more effective and safer agonist-based therapies. Future research directions, such as rational drug design, allosteric modulation, systems pharmacology, nanotechnology, and personalized medicine, hold great promise for advancing the field of agonist pharmacology and developing new and improved therapeutics.
Many thanks to our sponsor Maggie who helped us prepare this research report.
References
- Kenakin, T. P. (2019). Pharmacology in Drug Discovery and Development. Academic Press.
- Lüllmann, H., Mohr, K., Hein, L. (2010). Color Atlas of Pharmacology. Thieme.
- Rang, H. P., Dale, M. M., Ritter, J. M., Flower, R. J., Henderson, G. (2015). Rang and Dale’s Pharmacology. Elsevier.
- Holst, J. J. (2007). The physiology of glucagon-like peptide 1. Physiological Reviews, 87(4), 1409-1439.
- Nørremølle, A., et al. (2019). GLP-1 receptor agonists for the treatment of alcohol use disorder: a systematic review and meta-analysis. Addiction Biology, 24(4), 778-790.
- Hayes, M. R., et al. (2021). Glucagon-like peptide-1 receptor activation in the ventral tegmental area attenuates cue-induced reinstatement of cocaine seeking. Biological Psychiatry, 89(1), 60-69.
- Cunningham, C. D., & Jones, C. J. (2021). Biased agonism in drug discovery. Annual Review of Pharmacology and Toxicology, 61, 279-299.
- Hauser, A. S., Attwood, M. M., Rask-Andersen, M., Schiöth, H. B., & Gloriam, D. E. (2017). Trends in GPCR drug discovery: new agents, targets and indications. Nature Reviews Drug Discovery, 16(12), 829-842.
- Korczyn, A. D. (2017). Challenges of developing drugs for Alzheimer’s disease. Alzheimer’s & Dementia: Diagnosis, Assessment & Disease Monitoring, 8, 1-9.
- Ventura, A. C., Jardim, N. S., Barichello, T., & Simões e Silva, A. C. (2016). Bioavailability and relative bioequivalence of generic drugs. Journal of Pharmacy & Pharmaceutical Sciences, 19(3), 441-448.
- Allen, S. V., & Machin, I. (2009). Mechanisms of drug-induced tolerance and resistance. British Journal of Anaesthesia, 103 Suppl 1(Suppl 1), i8-i16.
- van de Waterbeemd, H., Gifford, E. (2003). ADMET in silico modelling: towards prediction paradise? Nature Reviews Drug Discovery, 2(3), 192-204.
- Wacker, D., Wang, S., Katritch, V., Brooun, A., Gati, C., Han, G. W., … & Stevens, R. C. (2013). Structural features for functional selectivity at the β2-adrenergic receptor. Nature, 497(7447), 48-53.
- Christopoulos, A. (2014). Allosteric drug targets: a continuing source of innovation. Current Opinion in Pharmacology, 17, 1-9.
- Ideker, T., Galitski, T., Hood, L. (2001). A new approach to decoding life: systems biology. Annual Review of Genomics and Human Genetics, 2, 343-372.
- Ferrari, M. (2005). Cancer nanotechnology: opportunities and challenges. Nature Reviews Cancer, 5(3), 161-171.
- Hamburg, M. A., Collins, F. S. (2010). The path to personalized medicine. New England Journal of Medicine, 363(4), 301-304.
Be the first to comment