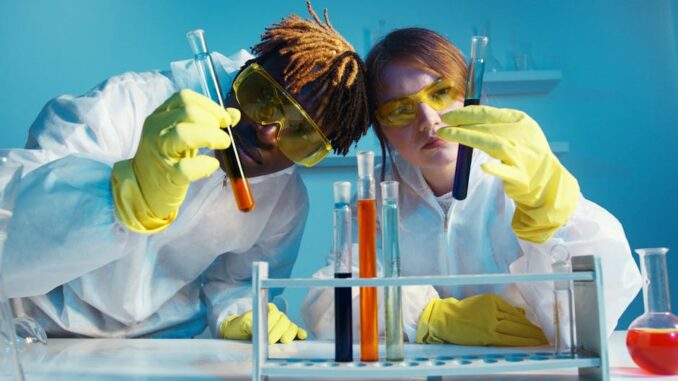
Abstract
Receptors are pivotal cellular components that mediate communication between cells and their environment. This report provides a comprehensive overview of receptor pharmacology, encompassing receptor structure, function, signaling mechanisms, and therapeutic implications. We delve into the diverse classes of receptors, including G protein-coupled receptors (GPCRs), receptor tyrosine kinases (RTKs), ligand-gated ion channels (LGICs), and nuclear receptors (NRs), examining their unique structural features and signaling pathways. Furthermore, we explore the intricate regulatory mechanisms that govern receptor expression, trafficking, and desensitization. The report also highlights the critical role of receptors in various physiological processes and pathological conditions, discussing their involvement in diseases such as cancer, neurological disorders, and cardiovascular diseases. Finally, we discuss the current therapeutic strategies that target receptors, including the development of agonists, antagonists, and allosteric modulators, as well as emerging approaches such as biased agonism and PROTACs (proteolysis-targeting chimeras). Understanding the complexities of receptor pharmacology is crucial for developing novel and effective treatments for a wide range of human diseases.
Many thanks to our sponsor Maggie who helped us prepare this research report.
1. Introduction
Cellular communication relies on the ability of cells to sense and respond to signals from their environment. Receptors, specialized proteins located on the cell surface or within the cell, play a central role in this process. These molecules bind to specific signaling molecules, known as ligands, initiating a cascade of intracellular events that ultimately lead to a cellular response. The study of receptors, their interactions with ligands, and the resulting downstream effects constitutes the field of receptor pharmacology. This field is of paramount importance in drug discovery, as many therapeutic agents exert their effects by targeting specific receptors.
Receptors are not simply passive binding sites; they are dynamic entities whose function is tightly regulated. Receptor expression levels, trafficking, and sensitivity can be modulated by a variety of factors, including extracellular signals, intracellular signaling pathways, and genetic factors. Dysregulation of receptor function is implicated in a wide range of diseases, making receptors attractive therapeutic targets.
This report provides a comprehensive overview of receptor pharmacology, covering various receptor classes, signaling pathways, regulatory mechanisms, and therapeutic applications. We will explore the structural features that define each receptor class, the signaling cascades they initiate, and the mechanisms that control their activity. Furthermore, we will discuss the role of receptors in various diseases and the therapeutic strategies that have been developed to target them. This report aims to provide an expert-level understanding of receptor pharmacology, highlighting the complexities and nuances of this crucial field.
Many thanks to our sponsor Maggie who helped us prepare this research report.
2. Receptor Classes: Structure and Function
Receptors can be broadly classified into four major classes based on their structure and signaling mechanisms: G protein-coupled receptors (GPCRs), receptor tyrosine kinases (RTKs), ligand-gated ion channels (LGICs), and nuclear receptors (NRs). Each class exhibits distinct structural features and initiates unique signaling cascades.
2.1 G Protein-Coupled Receptors (GPCRs)
GPCRs constitute the largest family of cell surface receptors in the human genome, playing a critical role in a wide array of physiological processes, including neurotransmission, hormone signaling, and immune responses [1]. Structurally, GPCRs are characterized by seven transmembrane alpha-helices connected by intracellular and extracellular loops. The extracellular loops are involved in ligand binding, while the intracellular loops interact with intracellular signaling proteins, primarily heterotrimeric G proteins.
Upon ligand binding, GPCRs undergo a conformational change that activates associated G proteins. G proteins are composed of three subunits: α, β, and γ. The α subunit binds to GDP in the inactive state and GTP in the active state. Upon activation, the α subunit dissociates from the βγ complex and both the α subunit and the βγ complex can interact with downstream effector proteins, such as adenylyl cyclase, phospholipase C, and ion channels. These effector proteins then modulate the levels of second messengers, such as cAMP, IP3, and DAG, which in turn activate protein kinases and other signaling molecules, leading to a cellular response. There are several classes of G proteins (Gs, Gi/o, Gq, G12/13), each coupled to different GPCRs and effector proteins, resulting in diverse signaling outcomes.
The versatility of GPCR signaling is further enhanced by the phenomenon of biased agonism. Biased agonists are ligands that preferentially activate certain signaling pathways over others. This selectivity allows for the development of drugs that target specific downstream effects of GPCR activation, potentially minimizing unwanted side effects [2].
2.2 Receptor Tyrosine Kinases (RTKs)
RTKs are transmembrane receptors that possess intrinsic tyrosine kinase activity. They play a crucial role in cell growth, differentiation, and survival [3]. Structurally, RTKs consist of an extracellular ligand-binding domain, a single transmembrane helix, and an intracellular kinase domain. Upon ligand binding, RTKs undergo dimerization or oligomerization, leading to autophosphorylation of tyrosine residues within the kinase domain. These phosphorylated tyrosine residues serve as docking sites for intracellular signaling proteins, such as SH2 domain-containing proteins.
The binding of signaling proteins to phosphorylated RTKs activates various downstream pathways, including the Ras-MAPK pathway, the PI3K-Akt pathway, and the STAT pathway. These pathways regulate gene expression, protein synthesis, and cell cycle progression. Dysregulation of RTK signaling is frequently observed in cancer, making RTKs important therapeutic targets. Several RTK inhibitors, such as imatinib and gefitinib, have been successfully developed for cancer treatment.
2.3 Ligand-Gated Ion Channels (LGICs)
LGICs, also known as ionotropic receptors, are transmembrane proteins that form ion-conducting pores. These receptors mediate rapid synaptic transmission in the nervous system [4]. Ligand binding to LGICs induces a conformational change that opens the pore, allowing ions to flow across the cell membrane. The resulting change in membrane potential can either excite or inhibit the postsynaptic neuron.
LGICs are typically composed of multiple subunits that assemble to form the functional channel. Examples of LGICs include the nicotinic acetylcholine receptor (nAChR), the γ-aminobutyric acid type A receptor (GABAAR), and the glutamate receptors (AMPA, NMDA, and kainate receptors). Each receptor type exhibits distinct ion selectivity and pharmacological properties. For example, nAChRs are permeable to cations, while GABAARs are permeable to chloride ions. LGICs are targets for a variety of drugs, including anesthetics, sedatives, and anticonvulsants.
2.4 Nuclear Receptors (NRs)
NRs are intracellular receptors that regulate gene transcription. They are activated by lipophilic ligands, such as steroid hormones, thyroid hormones, and fatty acids [5]. NRs are typically composed of an N-terminal activation function domain, a DNA-binding domain, and a ligand-binding domain. Upon ligand binding, NRs undergo a conformational change that allows them to bind to specific DNA sequences, known as hormone response elements (HREs), located in the promoter region of target genes.
NR binding to HREs recruits coactivator or corepressor proteins, which modulate chromatin structure and regulate gene transcription. NRs play a crucial role in development, metabolism, and reproduction. Dysregulation of NR signaling is implicated in various diseases, including cancer, diabetes, and osteoporosis. Selective estrogen receptor modulators (SERMs), such as tamoxifen, are used to treat breast cancer by selectively modulating the activity of the estrogen receptor.
Many thanks to our sponsor Maggie who helped us prepare this research report.
3. Receptor Signaling Pathways
Receptor activation initiates a complex cascade of intracellular signaling events that ultimately lead to a cellular response. These signaling pathways involve a variety of proteins, including kinases, phosphatases, GTPases, and second messengers. The specific signaling pathways activated by a receptor depend on the receptor class, the ligand bound, and the cellular context.
3.1 GPCR Signaling Pathways
GPCRs activate a diverse array of signaling pathways through their interaction with G proteins. As previously mentioned, different classes of G proteins (Gs, Gi/o, Gq, G12/13) couple to different GPCRs and effector proteins, resulting in distinct signaling outcomes. For example, activation of Gs stimulates adenylyl cyclase, leading to an increase in cAMP levels. cAMP activates protein kinase A (PKA), which phosphorylates various target proteins, including transcription factors. Activation of Gi/o inhibits adenylyl cyclase, leading to a decrease in cAMP levels. Gq activates phospholipase C (PLC), which hydrolyzes phosphatidylinositol 4,5-bisphosphate (PIP2) to generate inositol 1,4,5-trisphosphate (IP3) and diacylglycerol (DAG). IP3 releases calcium from intracellular stores, while DAG activates protein kinase C (PKC). G12/13 activates Rho GTPases, which regulate cytoskeletal dynamics and cell motility.
In addition to G protein-mediated signaling, GPCRs can also activate signaling pathways independently of G proteins. For example, some GPCRs can directly interact with and activate kinases, such as receptor tyrosine kinases (RTKs). This cross-talk between GPCRs and RTKs can lead to synergistic signaling effects.
3.2 RTK Signaling Pathways
RTKs activate several important signaling pathways, including the Ras-MAPK pathway, the PI3K-Akt pathway, and the STAT pathway. The Ras-MAPK pathway is activated by the binding of the adaptor protein Grb2 to phosphorylated RTKs. Grb2 recruits the guanine nucleotide exchange factor Sos, which activates Ras, a small GTPase. Activated Ras activates the MAP kinase cascade, leading to the activation of the transcription factors AP-1 and Elk-1. The PI3K-Akt pathway is activated by the binding of the PI3K regulatory subunit to phosphorylated RTKs. PI3K phosphorylates phosphatidylinositol 4,5-bisphosphate (PIP2) to generate phosphatidylinositol 3,4,5-trisphosphate (PIP3). PIP3 recruits the serine/threonine kinase Akt to the plasma membrane, where it is activated by PDK1 and mTORC2. Activated Akt phosphorylates various target proteins, including transcription factors and pro-apoptotic proteins. The STAT pathway is activated by the binding of STAT proteins to phosphorylated RTKs. STAT proteins are phosphorylated by the Janus kinases (JAKs), which are activated by RTK signaling. Phosphorylated STAT proteins dimerize and translocate to the nucleus, where they regulate gene transcription.
3.3 LGIC Signaling Pathways
LGICs mediate rapid synaptic transmission by allowing ions to flow across the cell membrane. The resulting change in membrane potential can either excite or inhibit the postsynaptic neuron. Excitatory LGICs, such as the AMPA and NMDA receptors, are permeable to cations, leading to depolarization of the postsynaptic neuron. Inhibitory LGICs, such as the GABAAR, are permeable to chloride ions, leading to hyperpolarization of the postsynaptic neuron. The activation of LGICs can also trigger intracellular signaling cascades. For example, activation of NMDA receptors leads to an influx of calcium ions, which can activate calcium-dependent signaling pathways.
3.4 NR Signaling Pathways
NRs regulate gene expression by binding to specific DNA sequences in the promoter region of target genes. Upon ligand binding, NRs recruit coactivator or corepressor proteins, which modulate chromatin structure and regulate gene transcription. Coactivators promote gene transcription by acetylating histones, while corepressors inhibit gene transcription by deacetylating histones. NRs can also interact with other transcription factors to regulate gene expression.
Many thanks to our sponsor Maggie who helped us prepare this research report.
4. Receptor Regulation and Desensitization
Receptor function is tightly regulated to ensure appropriate cellular responses to signaling molecules. Several mechanisms contribute to receptor regulation, including receptor expression, trafficking, and desensitization.
4.1 Receptor Expression
Receptor expression levels are controlled by a variety of factors, including gene transcription, mRNA stability, and protein degradation. The promoter region of receptor genes contains binding sites for transcription factors that can either increase or decrease receptor expression. mRNA stability is regulated by RNA-binding proteins and microRNAs. Protein degradation is mediated by the ubiquitin-proteasome system and the lysosomal pathway. Changes in receptor expression can significantly alter cellular sensitivity to signaling molecules.
4.2 Receptor Trafficking
Receptor trafficking involves the movement of receptors between different cellular compartments, including the endoplasmic reticulum, Golgi apparatus, plasma membrane, and endosomes. Receptor trafficking is regulated by various proteins, including adaptors, motors, and GTPases. Receptor trafficking is important for receptor maturation, localization, and internalization. Receptor internalization can lead to receptor degradation or receptor recycling back to the plasma membrane.
4.3 Receptor Desensitization
Receptor desensitization is a process by which cells reduce their responsiveness to a stimulus over time. Several mechanisms contribute to receptor desensitization, including receptor phosphorylation, receptor internalization, and receptor downregulation. Receptor phosphorylation is mediated by kinases, such as G protein-coupled receptor kinases (GRKs) and protein kinase C (PKC). Receptor phosphorylation can lead to receptor uncoupling from downstream signaling pathways and receptor internalization. Receptor internalization can lead to receptor degradation or receptor recycling back to the plasma membrane. Receptor downregulation involves a decrease in the total number of receptors due to decreased receptor synthesis or increased receptor degradation. Desensitization is a critical mechanism for preventing overstimulation and maintaining cellular homeostasis.
Many thanks to our sponsor Maggie who helped us prepare this research report.
5. Receptors in Disease and Therapy
Receptors play a crucial role in various physiological processes and are implicated in a wide range of diseases. Dysregulation of receptor function can contribute to the development and progression of diseases such as cancer, neurological disorders, and cardiovascular diseases. Consequently, receptors are attractive therapeutic targets, and many drugs exert their effects by targeting specific receptors.
5.1 Receptors in Cancer
RTKs are frequently overexpressed or mutated in cancer cells, leading to increased cell proliferation, survival, and metastasis [6]. Several RTK inhibitors, such as imatinib, gefitinib, and erlotinib, have been successfully developed for cancer treatment. These inhibitors block RTK activity, thereby inhibiting downstream signaling pathways and suppressing cancer cell growth. GPCRs also play a role in cancer development and progression. Some GPCRs promote cancer cell proliferation and metastasis, while others suppress cancer cell growth. NR signaling is also dysregulated in cancer. Selective estrogen receptor modulators (SERMs), such as tamoxifen, are used to treat breast cancer by selectively modulating the activity of the estrogen receptor.
5.2 Receptors in Neurological Disorders
LGICs play a critical role in synaptic transmission and are implicated in various neurological disorders, including epilepsy, Alzheimer’s disease, and Parkinson’s disease [7]. Mutations in LGIC genes can cause epilepsy. NMDA receptor antagonists are being investigated as potential treatments for Alzheimer’s disease. Dopamine receptors are involved in Parkinson’s disease, and drugs that increase dopamine levels or activate dopamine receptors are used to treat this disorder. GPCRs are also important in neurological disorders. Opioid receptors are targeted by analgesic drugs, and serotonin receptors are targeted by antidepressants and anti-anxiety drugs.
5.3 Receptors in Cardiovascular Diseases
GPCRs play a crucial role in regulating cardiovascular function and are implicated in various cardiovascular diseases, including hypertension, heart failure, and atherosclerosis [8]. β-adrenergic receptors are targeted by β-blockers, which are used to treat hypertension and heart failure. Angiotensin II receptors are targeted by angiotensin receptor blockers (ARBs), which are also used to treat hypertension and heart failure. RTKs are also involved in cardiovascular diseases. Vascular endothelial growth factor (VEGF) receptors are targeted by anti-angiogenic drugs, which are used to treat atherosclerosis.
5.4 Therapeutic Strategies Targeting Receptors
Several therapeutic strategies have been developed to target receptors, including the development of agonists, antagonists, and allosteric modulators. Agonists are ligands that activate receptors, mimicking the effects of the endogenous ligand. Antagonists are ligands that block receptor activation, preventing the endogenous ligand from binding and activating the receptor. Allosteric modulators are ligands that bind to a site on the receptor distinct from the ligand-binding site and modulate receptor activity. Allosteric modulators can either increase (positive allosteric modulators) or decrease (negative allosteric modulators) receptor activity. More recently, biased agonists, which selectively activate certain signaling pathways downstream of a receptor, have gained prominence as a way to refine drug targeting and minimise side effects. PROTACs (proteolysis-targeting chimeras) are a new class of therapeutics which bind to the target protein and an E3 ubiquitin ligase, leading to ubiquitination and degradation of the target protein. This method of targeted protein knockdown offers a new dimension to receptor therapeutics.
Many thanks to our sponsor Maggie who helped us prepare this research report.
6. Conclusion
Receptors are essential components of cellular communication, mediating the effects of a wide range of signaling molecules. This report has provided a comprehensive overview of receptor pharmacology, covering receptor structure, function, signaling mechanisms, and therapeutic implications. We have explored the diverse classes of receptors, including GPCRs, RTKs, LGICs, and NRs, examining their unique structural features and signaling pathways. Furthermore, we have discussed the intricate regulatory mechanisms that govern receptor expression, trafficking, and desensitization. The report has also highlighted the critical role of receptors in various physiological processes and pathological conditions, discussing their involvement in diseases such as cancer, neurological disorders, and cardiovascular diseases. Finally, we discussed the current therapeutic strategies that target receptors, including the development of agonists, antagonists, and allosteric modulators, as well as emerging approaches such as biased agonism and PROTACs. A thorough understanding of receptor pharmacology is crucial for developing novel and effective treatments for a wide range of human diseases. Future research should focus on elucidating the complex interactions between receptors and their signaling pathways, as well as developing more selective and effective receptor-targeted therapies. The continued development of novel approaches, such as biased agonism, PROTACs and gene therapy, hold great promise for revolutionizing the treatment of receptor-related diseases.
Many thanks to our sponsor Maggie who helped us prepare this research report.
References
[1] Rosenbaum, D. M., Rasmussen, S. G. F., & Kobilka, B. K. (2009). GPCR engineering for structure and function. Nature, 457(7225), 39-47.
[2] Kenakin, T. (2019). Functional selectivity and biased receptor signalling. Journal of Pharmacology and Experimental Therapeutics, 370(3), 674-681.
[3] Schlessinger, J. (2000). Cell signaling by receptor tyrosine kinases. Cell, 103(2), 211-225.
[4] Albuquerque, E. X., Pereira, E. F., Alkondon, M., & Rogers, S. W. (2009). Mammalian nicotinic acetylcholine receptors: from structure to function. Physiological Reviews, 89(1), 73-120.
[5] Tsai, M. J., & O’Malley, B. W. (1994). Molecular mechanisms of action of steroid/thyroid receptor superfamily members. Annual Review of Biochemistry, 63(1), 451-486.
[6] Arteaga, C. L. (2002). Overview of receptor tyrosine kinases in human cancer. Seminars in Oncology, 29(6 Suppl 18), 3-9.
[7] Macdonald, R. L., & Olsen, R. W. (1994). GABAA receptor channels. Annual Review of Neuroscience, 17(1), 569-602.
[8] Bristow, M. R. (2000). β-Adrenergic receptor blockade in chronic heart failure. Circulation, 101(5), 558-569.
[9] Gray, N. S., & Eksterowicz, J. (2021). Proteolysis-targeting chimeras (PROTACs) for targeted protein degradation in drug discovery. SLAS Discovery: Advancing Life Sciences R&D, 26(1), 39-55.
Be the first to comment