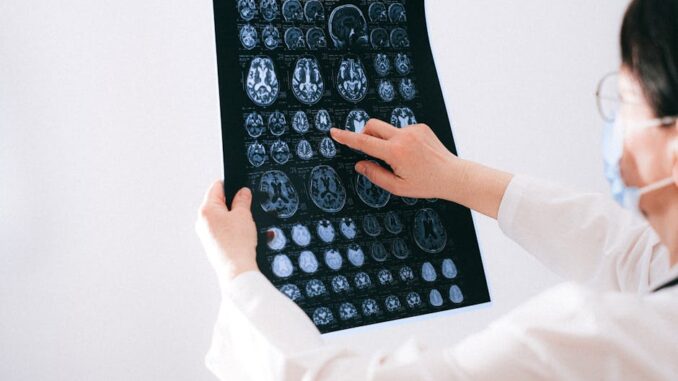
Neurotransmitters: Orchestrators of Brain Function and Vulnerability to Dysfunction
Abstract
Neurotransmitters, the chemical messengers of the brain, are fundamental to a vast array of physiological and psychological processes, ranging from basic motor control and sensory perception to complex cognitive functions, emotional regulation, and reward processing. This research report provides a comprehensive overview of neurotransmitters, encompassing their synthesis, release, receptor interactions, and inactivation mechanisms. It delves into the critical roles of key neurotransmitter systems, including glutamate, GABA, dopamine, serotonin, norepinephrine, acetylcholine, and endocannabinoids, highlighting their contributions to both normal brain function and various neurological and psychiatric disorders. Furthermore, the report explores the intricate interplay between neurotransmitter systems and the impact of factors such as stress, addiction, and genetic predispositions on neurotransmitter function. It concludes by discussing current and emerging therapeutic strategies aimed at modulating neurotransmitter activity to alleviate symptoms and improve outcomes in neurological and psychiatric conditions, and how these approaches can be tailored to individual patient profiles for more effective treatment.
Many thanks to our sponsor Maggie who helped us prepare this research report.
1. Introduction
The human brain, a remarkably complex organ, relies on a sophisticated communication network to coordinate its diverse functions. At the heart of this network are neurotransmitters, endogenous chemicals that transmit signals across synapses – the specialized junctions between neurons. These chemical messengers orchestrate a symphony of neuronal activity, enabling us to perceive the world, learn, remember, experience emotions, and execute movements. Dysregulation of neurotransmitter systems, whether due to genetic factors, environmental stressors, or disease processes, can disrupt this delicate balance and contribute to a wide range of neurological and psychiatric disorders.
This report aims to provide a comprehensive overview of neurotransmitters, exploring their synthesis, release, receptor interactions, and inactivation mechanisms. It will delve into the specific roles of major neurotransmitter systems, including glutamate, GABA, dopamine, serotonin, norepinephrine, acetylcholine, and endocannabinoids, and discuss how their dysfunction contributes to various neurological and psychiatric conditions. Furthermore, the report will examine the impact of factors such as stress, addiction, and genetic predispositions on neurotransmitter function, and explore therapeutic strategies aimed at modulating neurotransmitter activity to alleviate symptoms and improve outcomes.
Many thanks to our sponsor Maggie who helped us prepare this research report.
2. Neurotransmitter Synthesis, Release, and Receptor Interactions
2.1 Synthesis and Storage
Neurotransmitters are synthesized within neurons through a series of enzymatic reactions, often involving precursors derived from dietary sources. The specific synthetic pathways vary depending on the neurotransmitter. For example, dopamine, norepinephrine, and epinephrine are synthesized from the amino acid tyrosine through a cascade of enzymatic steps involving tyrosine hydroxylase (TH), aromatic L-amino acid decarboxylase (AADC), dopamine-beta-hydroxylase (DBH), and phenylethanolamine N-methyltransferase (PNMT). Serotonin, on the other hand, is synthesized from tryptophan through the action of tryptophan hydroxylase (TPH) and AADC. Glutamate, the primary excitatory neurotransmitter, can be synthesized from glutamine by glutaminase, or as a byproduct of the Krebs cycle.
Following synthesis, neurotransmitters are typically stored within synaptic vesicles, small membrane-bound organelles located in the presynaptic terminal. Vesicular storage protects neurotransmitters from degradation and ensures their availability for rapid release upon neuronal stimulation. Vesicular monoamine transporter 2 (VMAT2) plays a critical role in transporting monoamine neurotransmitters (dopamine, norepinephrine, serotonin) into vesicles, while vesicular glutamate transporters (VGLUTs) and vesicular GABA transporters (VGAT) mediate the uptake of glutamate and GABA, respectively.
2.2 Release and Diffusion
Neurotransmitter release is a tightly regulated process triggered by the arrival of an action potential at the presynaptic terminal. Depolarization of the presynaptic membrane opens voltage-gated calcium channels, allowing calcium ions to flow into the terminal. The influx of calcium triggers the fusion of synaptic vesicles with the presynaptic membrane, releasing their contents into the synaptic cleft – the narrow space between the pre- and postsynaptic neurons. This process, known as exocytosis, is mediated by a complex of proteins including SNAREs (soluble N-ethylmaleimide-sensitive factor attachment protein receptors).
Once released into the synaptic cleft, neurotransmitters diffuse rapidly across the synapse to reach the postsynaptic membrane. The concentration of neurotransmitters in the cleft is transient, as they are rapidly cleared by reuptake transporters, enzymatic degradation, or diffusion away from the synapse.
2.3 Receptor Binding and Postsynaptic Effects
Neurotransmitters exert their effects by binding to specific receptors located on the postsynaptic neuron. Neurotransmitter receptors can be broadly classified into two main types: ionotropic receptors and metabotropic receptors.
Ionotropic receptors are ligand-gated ion channels that directly allow ions to flow across the postsynaptic membrane upon neurotransmitter binding. This ion flux can lead to depolarization (excitation) or hyperpolarization (inhibition) of the postsynaptic neuron. Examples of ionotropic receptors include the AMPA, NMDA, and kainate receptors for glutamate, the GABAA receptor for GABA, and the nicotinic acetylcholine receptor.
Metabotropic receptors, also known as G protein-coupled receptors (GPCRs), do not directly gate ion channels. Instead, they activate intracellular signaling cascades through the activation of G proteins. G proteins can modulate the activity of various effector proteins, including enzymes that produce second messengers such as cAMP or IP3, or ion channels that can directly affect neuronal excitability. Metabotropic receptors offer a greater diversity of signaling mechanisms compared to ionotropic receptors and can produce longer-lasting effects on neuronal function. Examples of metabotropic receptors include the metabotropic glutamate receptors (mGluRs), GABAB receptors, dopamine receptors (D1-D5), serotonin receptors (5-HT1-5-HT7), adrenergic receptors (alpha and beta), and muscarinic acetylcholine receptors.
The specific postsynaptic effects of a neurotransmitter depend on the type of receptor it binds to and the intracellular signaling pathways that are activated. For example, activation of AMPA receptors by glutamate typically leads to rapid depolarization of the postsynaptic neuron, while activation of GABAB receptors by GABA can lead to hyperpolarization through the opening of potassium channels.
2.4 Neurotransmitter Inactivation
The signaling by neurotransmitters needs to be tightly controlled, therefore the processes of inactivation are very important. Neurotransmitters are removed from the synaptic cleft through several mechanisms, including reuptake, enzymatic degradation, and diffusion.
Reuptake involves the transport of neurotransmitters back into the presynaptic neuron or surrounding glial cells by specific transporter proteins. These transporters effectively clear the neurotransmitter from the synapse, preventing its continued activation of postsynaptic receptors. Examples of reuptake transporters include the dopamine transporter (DAT), the serotonin transporter (SERT), and the norepinephrine transporter (NET).
Enzymatic degradation involves the breakdown of neurotransmitters by specific enzymes located in the synaptic cleft or within neurons. For example, acetylcholinesterase (AChE) rapidly hydrolyzes acetylcholine into choline and acetate, terminating its signaling. Monoamine oxidase (MAO) and catechol-O-methyltransferase (COMT) are involved in the degradation of monoamine neurotransmitters (dopamine, norepinephrine, serotonin).
Diffusion involves the movement of neurotransmitters away from the synapse, reducing their concentration in the synaptic cleft. This process is less efficient than reuptake or enzymatic degradation, but it can contribute to the overall clearance of neurotransmitters from the synapse.
Many thanks to our sponsor Maggie who helped us prepare this research report.
3. Key Neurotransmitter Systems and Their Functions
3.1 Glutamate
Glutamate is the primary excitatory neurotransmitter in the central nervous system, playing a critical role in synaptic plasticity, learning, memory, and neuronal development. It is estimated that over half of all synapses in the brain use glutamate as their neurotransmitter. Glutamate acts on both ionotropic receptors (AMPA, NMDA, and kainate receptors) and metabotropic receptors (mGluRs). Imbalances in glutamate neurotransmission have been implicated in a wide range of neurological disorders, including epilepsy, stroke, traumatic brain injury, and neurodegenerative diseases such as Alzheimer’s and Parkinson’s diseases. Excessive glutamate activity, known as excitotoxicity, can lead to neuronal damage and cell death. The NMDA receptor is particularly important for synaptic plasticity and learning, but also contributes significantly to excitotoxicity when overstimulated. There is a significant amount of research looking into the development of drugs that act on the NMDA receptor for use in a wide range of disorders, but the potential for side effects is limiting.
3.2 GABA
GABA (gamma-aminobutyric acid) is the primary inhibitory neurotransmitter in the brain, counterbalancing the excitatory effects of glutamate. GABAergic neurons are widely distributed throughout the brain and play a critical role in regulating neuronal excitability, anxiety, sleep, and muscle tone. GABA acts on both ionotropic (GABAA) and metabotropic (GABAB) receptors. GABAA receptors are ligand-gated chloride channels that mediate fast inhibitory neurotransmission, while GABAB receptors are G protein-coupled receptors that mediate slower and more prolonged inhibitory effects. Dysfunction of GABAergic neurotransmission has been implicated in anxiety disorders, insomnia, epilepsy, and schizophrenia. Drugs that enhance GABA activity, such as benzodiazepines, are commonly used to treat anxiety and insomnia. The discovery of numerous GABAA receptor subtypes adds complexity, offering potential targets for more selective anxiolytics with fewer side effects.
3.3 Dopamine
Dopamine is a monoamine neurotransmitter involved in a variety of functions, including reward, motivation, motor control, cognition, and emotion. Dopaminergic neurons are primarily located in the midbrain regions of the substantia nigra and the ventral tegmental area (VTA). The dopaminergic pathways originating from the substantia nigra project to the dorsal striatum and are critical for motor control. Degeneration of these neurons leads to Parkinson’s disease. The dopaminergic pathways originating from the VTA project to the nucleus accumbens, prefrontal cortex, and amygdala and are involved in reward processing, motivation, and cognitive function. Dopamine acts on five different subtypes of G protein-coupled receptors (D1-D5). Dysfunction of dopaminergic neurotransmission has been implicated in Parkinson’s disease, schizophrenia, addiction, and attention-deficit/hyperactivity disorder (ADHD). Drugs that increase dopamine levels, such as stimulants, are commonly used to treat ADHD, while drugs that block dopamine receptors, such as antipsychotics, are used to treat schizophrenia. The role of dopamine in reward and reinforcement is pivotal to understanding addictive behaviors, where dopamine release reinforces drug-seeking behavior.
3.4 Serotonin
Serotonin (5-hydroxytryptamine, 5-HT) is a monoamine neurotransmitter involved in mood regulation, sleep, appetite, and cognition. Serotonergic neurons are primarily located in the raphe nuclei in the brainstem and project widely throughout the brain. Serotonin acts on at least 14 different subtypes of receptors, most of which are G protein-coupled receptors. Dysfunction of serotonergic neurotransmission has been implicated in depression, anxiety disorders, obsessive-compulsive disorder (OCD), and migraine. Selective serotonin reuptake inhibitors (SSRIs), which block the reuptake of serotonin, are commonly used to treat depression and anxiety disorders. Research increasingly points to the role of gut microbiota in serotonin production and regulation, adding another layer of complexity to understanding its role in mental health.
3.5 Norepinephrine
Norepinephrine (noradrenaline) is a monoamine neurotransmitter involved in alertness, arousal, attention, and the fight-or-flight response. Noradrenergic neurons are primarily located in the locus coeruleus in the brainstem and project widely throughout the brain. Norepinephrine acts on alpha and beta adrenergic receptors, both of which are G protein-coupled receptors. Dysfunction of noradrenergic neurotransmission has been implicated in depression, anxiety disorders, and ADHD. Drugs that increase norepinephrine levels, such as norepinephrine reuptake inhibitors (NRIs), are used to treat depression and ADHD. Dysregulation of norepinephrine systems is intricately linked to stress response and can impact cognitive functions like working memory and attention.
3.6 Acetylcholine
Acetylcholine is a neurotransmitter involved in motor control, cognition, and memory. Cholinergic neurons are located in various brain regions, including the basal forebrain and the brainstem. Acetylcholine acts on two main types of receptors: nicotinic acetylcholine receptors, which are ionotropic receptors, and muscarinic acetylcholine receptors, which are G protein-coupled receptors. Dysfunction of cholinergic neurotransmission has been implicated in Alzheimer’s disease and myasthenia gravis. Drugs that increase acetylcholine levels, such as cholinesterase inhibitors, are used to treat Alzheimer’s disease. The role of acetylcholine in attention and wakefulness is also being actively explored, with implications for understanding sleep disorders and cognitive deficits.
3.7 Endocannabinoids
Endocannabinoids are endogenous lipid-based neurotransmitters that bind to cannabinoid receptors (CB1 and CB2). CB1 receptors are primarily located in the brain and are involved in regulating mood, pain, appetite, and memory. CB2 receptors are primarily located in the immune system and are involved in regulating inflammation and immune responses. Endocannabinoids are synthesized on demand and released from the postsynaptic neuron, acting as retrograde messengers to modulate presynaptic neurotransmitter release. Dysfunction of the endocannabinoid system has been implicated in anxiety disorders, depression, and chronic pain. The interaction between the endocannabinoid system and other neurotransmitter systems is complex, impacting various functions from stress response to neuroprotection. Furthermore, the potential therapeutic uses of compounds targeting the endocannabinoid system are being extensively researched, but require careful consideration of possible side effects and regulatory frameworks.
Many thanks to our sponsor Maggie who helped us prepare this research report.
4. The Interplay Between Neurotransmitter Systems
The different neurotransmitter systems do not operate in isolation; they interact extensively to regulate brain function. These interactions can be synergistic, antagonistic, or modulatory. For example, glutamate and GABA exert opposing effects on neuronal excitability, with glutamate promoting excitation and GABA promoting inhibition. The balance between these two neurotransmitter systems is critical for maintaining neuronal homeostasis. Dopamine and serotonin interact in complex ways to regulate mood, motivation, and reward processing. Serotonin can modulate dopamine release in the striatum, influencing reward-related behaviors. Norepinephrine can modulate the activity of both glutamate and GABA neurons, affecting attention, arousal, and stress responses. Acetylcholine interacts with dopamine in the basal ganglia to regulate motor control and with glutamate in the hippocampus to regulate memory.
The interactions between neurotransmitter systems can be disrupted by various factors, including genetic predispositions, environmental stressors, and drug use. Such disruptions can contribute to the development of neurological and psychiatric disorders. For example, chronic stress can alter the balance between glutamate and GABA, leading to anxiety and depression. Drug abuse can disrupt dopaminergic neurotransmission, leading to addiction. Understanding the complex interplay between neurotransmitter systems is crucial for developing effective treatments for neurological and psychiatric disorders.
Many thanks to our sponsor Maggie who helped us prepare this research report.
5. Factors Affecting Neurotransmitter Function
5.1 Stress
Stress has a profound impact on neurotransmitter function. Acute stress can lead to increased release of norepinephrine, dopamine, and glutamate, preparing the organism for a fight-or-flight response. Chronic stress, however, can disrupt the balance of neurotransmitter systems, leading to anxiety, depression, and cognitive impairment. Chronic stress can decrease serotonin and dopamine levels, while increasing glutamate levels. These changes can alter synaptic plasticity and neuronal morphology, leading to long-lasting effects on brain function. The hypothalamic-pituitary-adrenal (HPA) axis, a key component of the stress response system, plays a critical role in mediating the effects of stress on neurotransmitter function. Cortisol, the primary stress hormone released by the adrenal glands, can modulate the activity of various neurotransmitter systems, including glutamate, GABA, dopamine, and serotonin.
5.2 Addiction
Addiction is characterized by compulsive drug-seeking and drug-taking behavior despite negative consequences. Drugs of abuse hijack the brain’s reward circuitry, primarily by increasing dopamine levels in the nucleus accumbens. Chronic drug use can lead to long-lasting changes in the dopaminergic system, including decreased dopamine receptor density and impaired dopamine release. These changes can contribute to the development of tolerance, dependence, and withdrawal symptoms. Drug use also affects other neurotransmitter systems, including glutamate, GABA, serotonin, and norepinephrine. For example, alcohol enhances GABA activity and inhibits glutamate activity, leading to sedation and impaired cognitive function. Opioids activate opioid receptors, leading to analgesia and euphoria, but also suppress GABA release, which can contribute to respiratory depression. The complexity of neurotransmitter involvement in addiction highlights the challenges in developing effective treatments. Understanding the neurobiological mechanisms underlying addiction is crucial for developing effective prevention and treatment strategies. Targeted therapies that modulate specific neurotransmitter systems or promote neuroplasticity are showing promise in preclinical and clinical studies.
5.3 Genetic Predispositions
Genetic factors play a significant role in determining individual differences in neurotransmitter function and susceptibility to neurological and psychiatric disorders. Genes involved in neurotransmitter synthesis, release, receptor expression, and inactivation can influence the activity of neurotransmitter systems. For example, variations in the genes encoding dopamine receptors (e.g., DRD2, DRD4) have been associated with individual differences in reward sensitivity and impulsivity. Variations in the genes encoding serotonin transporters (e.g., SLC6A4) have been associated with individual differences in anxiety and depression. Genome-wide association studies (GWAS) have identified numerous genetic variants associated with neurological and psychiatric disorders, many of which are involved in neurotransmitter function. Epigenetic mechanisms, such as DNA methylation and histone modification, can also influence neurotransmitter function by altering gene expression. Understanding the genetic and epigenetic factors that influence neurotransmitter function is crucial for developing personalized approaches to prevention and treatment of neurological and psychiatric disorders.
Many thanks to our sponsor Maggie who helped us prepare this research report.
6. Therapeutic Strategies for Modulating Neurotransmitter Activity
6.1 Pharmacological Interventions
Pharmacological interventions are a mainstay of treatment for many neurological and psychiatric disorders. These interventions typically target specific neurotransmitter systems to alleviate symptoms and improve function. For example, SSRIs are used to treat depression and anxiety disorders by blocking the reuptake of serotonin. Antipsychotics are used to treat schizophrenia by blocking dopamine receptors. Benzodiazepines are used to treat anxiety and insomnia by enhancing GABA activity. Cholinesterase inhibitors are used to treat Alzheimer’s disease by increasing acetylcholine levels. While these drugs can be effective, they often have side effects and may not be effective for all patients. Furthermore, long-term use can lead to tolerance and dependence. Developing more selective and targeted pharmacological interventions is an ongoing area of research. This includes developing drugs that target specific receptor subtypes or intracellular signaling pathways. An example of this is the evolving treatment of depression, where research is increasingly focused on novel targets such as the NMDA receptor and inflammatory pathways, alongside traditional serotonergic mechanisms.
6.2 Non-Pharmacological Therapies
Non-pharmacological therapies, such as psychotherapy, cognitive behavioral therapy (CBT), and neuromodulation techniques, can also be effective in modulating neurotransmitter activity and improving outcomes in neurological and psychiatric disorders. Psychotherapy and CBT can help patients develop coping skills and change maladaptive thought patterns and behaviors, which can indirectly affect neurotransmitter function. Neuromodulation techniques, such as transcranial magnetic stimulation (TMS) and deep brain stimulation (DBS), can directly modulate neuronal activity and neurotransmitter release in specific brain regions. TMS uses magnetic pulses to stimulate or inhibit neuronal activity in the cerebral cortex, while DBS involves implanting electrodes into specific brain regions to deliver electrical stimulation. These techniques are being increasingly used to treat depression, OCD, Parkinson’s disease, and other neurological and psychiatric disorders. Additionally, lifestyle interventions such as exercise, healthy diet, and stress reduction techniques can also have a positive impact on neurotransmitter function and overall mental health. For instance, regular exercise has been shown to increase levels of serotonin, dopamine, and norepinephrine. The synergistic effects of combining pharmacological and non-pharmacological therapies are also increasingly recognized as providing the most effective treatment strategy for many disorders.
6.3 Personalized Medicine Approaches
Personalized medicine approaches, which take into account individual genetic predispositions, environmental factors, and disease characteristics, are becoming increasingly important in the treatment of neurological and psychiatric disorders. These approaches aim to tailor treatment strategies to the specific needs of each patient, maximizing efficacy and minimizing side effects. Genetic testing can be used to identify individuals who are more likely to respond to certain medications or who are at higher risk for developing certain side effects. Neuroimaging techniques, such as fMRI and PET, can be used to assess brain function and neurotransmitter activity, providing valuable information for treatment planning. Integrating these data with clinical information can lead to more effective and targeted treatments. The future of neuropsychopharmacology lies in the development of precision medicine approaches that can predict treatment response and optimize therapeutic outcomes for individual patients.
Many thanks to our sponsor Maggie who helped us prepare this research report.
7. Conclusion
Neurotransmitters are fundamental to brain function, orchestrating a complex symphony of neuronal activity that enables us to perceive the world, learn, remember, and experience emotions. Dysregulation of neurotransmitter systems contributes to a wide range of neurological and psychiatric disorders. Understanding the intricate mechanisms of neurotransmitter synthesis, release, receptor interactions, and inactivation, as well as the interplay between different neurotransmitter systems, is crucial for developing effective treatments for these disorders. Factors such as stress, addiction, and genetic predispositions can significantly impact neurotransmitter function. Therapeutic strategies aimed at modulating neurotransmitter activity, including pharmacological interventions, non-pharmacological therapies, and personalized medicine approaches, hold great promise for alleviating symptoms and improving outcomes in neurological and psychiatric conditions. Further research is needed to fully elucidate the complex interactions between neurotransmitter systems and to develop more selective and targeted therapies that can address the specific needs of individual patients. The ongoing advancements in neuroscience and technology are paving the way for a future where personalized medicine approaches will revolutionize the treatment of neurological and psychiatric disorders.
Many thanks to our sponsor Maggie who helped us prepare this research report.
References
- Andersen, S. L. (2003). Trajectories of brain development: point of vulnerability or window of opportunity?. Neuroscience & Biobehavioral Reviews, 27(1-2), 3-18.
- Beaulieu, J. M., & Gainetdinov, R. R. (2011). The physiology, signaling, and pharmacology of dopamine receptors. Pharmacological Reviews, 63(1), 182-217.
- Blum, K., et al. (2012). Reward deficiency syndrome. Progress in Brain Research, 196, 107-148.
- Cooper, J. R., Bloom, F. E., & Roth, R. H. (2003). The Biochemical Basis of Neuropharmacology. Oxford University Press.
- Cryan, J. F., & Slattery, D. A. (2007). Stress and the HPA axis: insights from preclinical studies. Neuron, 55(6), 816-823.
- Duman, R. S. (2002). Synaptic plasticity and mood disorders. Biological Psychiatry, 52(7), 732-739.
- Elsworth, J. D., & Roth, R. H. (1997). Dopamine synthesis, storage, metabolism, and release. In Dopamine (pp. 149-173). Springer, Boston, MA.
- Goddard, A. W., et al. (2001). Serotonin function in generalized anxiety disorder. Biological Psychiatry, 49(10), 865-872.
- Hyman, S. E., Malenka, R. C., & Nestler, E. J. (2006). Neural mechanisms of addiction: the role of reward-related learning and memory. Annual Review of Neuroscience, 29, 565-598.
- Kandel, E. R., Schwartz, J. H., & Jessell, T. M. (2000). Principles of Neural Science. McGraw-Hill.
- Koob, G. F., & Volkow, N. D. (2016). Neurobiology of addiction: a neurocircuitry analysis. The Lancet Psychiatry, 3(8), 760-773.
- Lüscher, C., & Malenka, R. C. (2011). Drug-evoked synaptic plasticity in addiction: long-term potentiation, long-term depression, and beyond. Neuron, 69(4), 650-663.
- Nestler, E. J. (2005). Is there a common molecular pathway for addiction?. Nature Neuroscience, 8(11), 1445-1449.
- Nutt, D. J. (2008). Relationship of neurotransmitters to the symptoms of major depressive disorder. The Journal of Clinical Psychiatry, 69 Suppl E1, 4-7.
- Stahl, S. M. (2013). Stahl’s Essential Psychopharmacology: Neuroscientific Basis and Practical Applications. Cambridge University Press.
- Volkow, N. D., Wang, G. J., Fowler, J. S., Tomasi, D., & Baler, R. (2010). Addiction: decreased reward sensitivity and increased expectation/motivation drive the shift from recreation to compulsion. Biological Psychiatry, 69(6), 499-506.
Be the first to comment