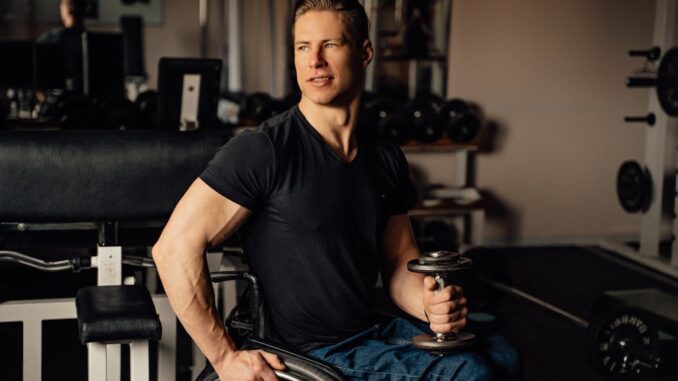
Abstract
Endorphins, endogenous opioid neuropeptides, have long been associated with the “runner’s high” and pain modulation. However, their physiological roles extend far beyond exercise-induced analgesia and euphoria. This research report provides a comprehensive overview of endorphins, delving into their neurobiological mechanisms, diverse physiological effects, and therapeutic potential across various clinical domains. We explore the biosynthesis, receptor interactions, and signaling pathways of endorphins, highlighting their involvement in stress response, immune modulation, social behavior, and reward circuitry. Furthermore, we critically analyze the evidence supporting the use of endorphin-releasing interventions, such as exercise, acupuncture, and mindfulness-based practices, in the management of pain, addiction, mood disorders, and neurodegenerative diseases. We also address the limitations of current research and propose future directions for unraveling the complex roles of endorphins in health and disease.
Many thanks to our sponsor Maggie who helped us prepare this research report.
1. Introduction
The discovery of endogenous opioid peptides, collectively known as endorphins, revolutionized our understanding of pain perception and the brain’s inherent capacity for self-regulation. Initially identified in the context of acupuncture-induced analgesia (Mayer et al., 1977), endorphins are now recognized as key players in a wide array of physiological processes, ranging from stress response and immune modulation to social behavior and reward circuitry (Bodnar, 2016). The term “endorphin” itself is a portmanteau of “endogenous morphine,” reflecting their structural similarity to exogenous opioid drugs and their ability to bind to opioid receptors, thereby eliciting analgesic and euphoric effects (Hughes, 1975). While the association between endorphins and exercise-induced euphoria, often referred to as the “runner’s high,” is widely acknowledged, this represents only a small facet of their multifaceted roles. This research report aims to provide a more in-depth exploration of endorphins, encompassing their neurobiological mechanisms, diverse physiological effects, and therapeutic potential across various clinical domains.
Many thanks to our sponsor Maggie who helped us prepare this research report.
2. Neurobiology of Endorphins
2.1 Biosynthesis and Processing
Endorphins are derived from larger precursor proteins, primarily proopiomelanocortin (POMC), which is synthesized in the pituitary gland, hypothalamus, and other brain regions (Nakanishi et al., 1979). POMC undergoes tissue-specific post-translational processing, resulting in the production of various bioactive peptides, including β-endorphin, α-melanocyte-stimulating hormone (α-MSH), adrenocorticotropic hormone (ACTH), and γ-lipotropin (γ-LPH) (Smith & Funder, 1988). β-endorphin, the most extensively studied endorphin, contains 31 amino acids and is a potent agonist at μ-opioid receptors (MORs) (Akil et al., 1984). Other endorphins, such as enkephalins and dynorphins, are derived from different precursor proteins (proenkephalin and prodynorphin, respectively) and exhibit varying affinities for different opioid receptor subtypes (MOR, δ-opioid receptor (DOR), and κ-opioid receptor (KOR)). The differential processing of POMC and other opioid precursors allows for a fine-tuned regulation of opioid signaling in different brain regions and under varying physiological conditions.
2.2 Opioid Receptors and Signaling Pathways
Endorphins exert their effects by binding to opioid receptors, which are G protein-coupled receptors (GPCRs) widely distributed throughout the central and peripheral nervous systems (Satoh & Minami, 1995). The three major opioid receptor subtypes – MOR, DOR, and KOR – exhibit distinct pharmacological profiles and mediate different physiological effects. MORs are primarily responsible for analgesia, euphoria, respiratory depression, and addiction (Pert & Snyder, 1973). DORs are involved in analgesia, mood regulation, and immune modulation (Knapp et al., 1996). KORs are associated with dysphoria, sedation, and antinociception (Vonvoigtlander et al., 1983). Activation of opioid receptors leads to the activation of intracellular signaling pathways, including the inhibition of adenylyl cyclase, the activation of potassium channels, and the inhibition of calcium channels (North, 1992). These signaling events ultimately result in a decrease in neuronal excitability and the modulation of synaptic transmission. Furthermore, opioid receptors can undergo desensitization and internalization upon prolonged exposure to agonists, leading to tolerance and dependence (Williams et al., 2013).
2.3 Regulation of Endorphin Release
The release of endorphins is tightly regulated by various factors, including stress, pain, exercise, and social interaction (Millan, 2002). Stressful stimuli, such as physical trauma, psychological distress, and social isolation, can trigger the release of endorphins, leading to analgesia and stress reduction (Basbaum & Fields, 1978). Exercise, particularly high-intensity aerobic exercise, has been shown to increase endorphin levels in the brain and periphery (Boecker et al., 2008). The mechanisms underlying exercise-induced endorphin release are complex and likely involve the activation of the hypothalamic-pituitary-adrenal (HPA) axis, the stimulation of peripheral afferent fibers, and the modulation of neurotransmitter systems (e.g., dopamine, serotonin) (Goldfarb & Jamurtas, 1997). Social interaction, particularly positive social interaction, can also stimulate endorphin release, contributing to feelings of social connectedness and well-being (Nummenmaa et al., 2016). The release of endorphins is also subject to feedback regulation, with opioid receptors located on endorphin-releasing neurons modulating their own activity (Mansour et al., 1994).
Many thanks to our sponsor Maggie who helped us prepare this research report.
3. Physiological Effects of Endorphins
3.1 Pain Modulation
The most well-established function of endorphins is their role in pain modulation. Endorphins can inhibit pain transmission at multiple levels of the nervous system, including the spinal cord, brainstem, and cortex (Basbaum & Fields, 1978). They activate descending inhibitory pathways that suppress nociceptive signals, reducing the perception of pain (Fields, 2004). Endorphins also modulate the release of pro-inflammatory cytokines, thereby reducing inflammation and pain associated with tissue injury (Kavelaars et al., 2000). The analgesic effects of endorphins are mediated primarily by MORs, but DORs and KORs also contribute to pain modulation under certain conditions (Ossipov et al., 2010). The endogenous opioid system plays a critical role in regulating pain in both acute and chronic pain states, and dysfunction of this system may contribute to the development and maintenance of chronic pain conditions.
3.2 Stress Response
Endorphins are integral to the body’s response to stress. During stressful situations, the HPA axis is activated, leading to the release of cortisol and endorphins (Guillemin et al., 1977). Cortisol mobilizes energy reserves and suppresses immune function, while endorphins counteract the aversive effects of stress by reducing pain, anxiety, and negative mood (Arborelius et al., 1999). Endorphins also modulate the activity of the autonomic nervous system, promoting relaxation and reducing heart rate and blood pressure (Lightman, 2008). Chronic stress can lead to dysregulation of the endogenous opioid system, resulting in decreased endorphin release and increased sensitivity to pain and stress (McEwen, 1998). Conversely, interventions that enhance endorphin release, such as exercise and mindfulness-based practices, can improve stress resilience and reduce the negative consequences of chronic stress.
3.3 Immune Modulation
Endorphins interact with the immune system, influencing immune cell function and cytokine production (Lysle et al., 2004). Opioid receptors are expressed on immune cells, including lymphocytes, macrophages, and natural killer cells, and activation of these receptors can modulate immune cell activity (Carr & Sergerstrom, 1993). Endorphins can enhance natural killer cell cytotoxicity, promote lymphocyte proliferation, and modulate the release of cytokines, such as interleukin-2 (IL-2) and interferon-gamma (IFN-γ) (Petitto et al., 1991). The effects of endorphins on the immune system are complex and can vary depending on the type of immune cell, the concentration of endorphins, and the presence of other immune mediators. In general, endorphins tend to enhance cellular immunity and suppress humoral immunity (Weber et al., 1987). Dysregulation of the endogenous opioid system has been implicated in the pathogenesis of various immune disorders, including autoimmune diseases and infectious diseases.
3.4 Social Behavior
Emerging evidence suggests that endorphins play a critical role in social behavior, particularly in the formation and maintenance of social bonds (Panksepp, 1998). Endorphins are released during social interaction, promoting feelings of social connectedness, trust, and empathy (Nummenmaa et al., 2016). Blocking opioid receptors can impair social bonding and increase social anxiety (Moles et al., 2004). Endorphins also contribute to the rewarding aspects of social interaction, reinforcing social behaviors and promoting social affiliation (Insel, 2010). Dysfunction of the endogenous opioid system has been implicated in social deficits associated with autism spectrum disorder and other social disorders (Panksepp & Lahvis, 2007). Targeted interventions aimed at enhancing endorphin release may hold promise for improving social function in individuals with social deficits.
3.5 Reward and Motivation
Endorphins are an integral component of the brain’s reward circuitry, contributing to feelings of pleasure, motivation, and reinforcement (Wise, 2002). Endorphins are released in response to rewarding stimuli, such as food, sex, and social interaction, activating MORs in the ventral tegmental area (VTA) and nucleus accumbens (NAc) (Di Chiara, 1998). Activation of MORs in these brain regions increases dopamine release, which is a key neurotransmitter involved in reward and motivation (Koob & Volkow, 2010). Endorphins also modulate the activity of other neurotransmitter systems involved in reward, such as serotonin and endocannabinoids (Bloom, 1996). Dysfunction of the endogenous opioid system has been implicated in the pathogenesis of addiction, as addictive drugs hijack the brain’s reward circuitry and lead to excessive endorphin release, resulting in dependence and withdrawal (Koob & Le Moal, 2001).
Many thanks to our sponsor Maggie who helped us prepare this research report.
4. Therapeutic Potential of Endorphins
4.1 Pain Management
The analgesic properties of endorphins have led to the development of various non-pharmacological approaches to pain management that aim to enhance endorphin release. These approaches include exercise therapy, acupuncture, transcutaneous electrical nerve stimulation (TENS), and mindfulness-based practices (Sluka & Walsh, 2003). Exercise therapy has been shown to be effective in reducing pain in various chronic pain conditions, such as fibromyalgia, osteoarthritis, and low back pain (Geneen et al., 2017). Acupuncture and TENS stimulate the release of endorphins, providing pain relief through activation of the endogenous opioid system (Han, 2004). Mindfulness-based practices, such as meditation and yoga, can also increase endorphin levels and reduce pain perception (Reiner et al., 2013). These non-pharmacological approaches offer a safe and effective alternative to opioid medications for the management of chronic pain.
4.2 Addiction Recovery
Endorphins play a crucial role in addiction recovery. Addiction is characterized by dysregulation of the brain’s reward circuitry, leading to decreased endorphin release and increased sensitivity to stress and negative emotions (Koob & Le Moal, 2001). Exercise therapy and other endorphin-releasing interventions can help restore the balance of the endogenous opioid system and reduce cravings and withdrawal symptoms (Brown et al., 2008). Exercise has been shown to increase endorphin levels in individuals recovering from opioid addiction, reducing anxiety, depression, and relapse rates (Weinhold et al., 2010). Furthermore, social support and participation in group activities can also enhance endorphin release, promoting social connectedness and reducing social isolation, which are major risk factors for relapse (Kelly et al., 2011). The opioid system’s role in social attachment and reward is pivotal to the success of 12-step and community reinforcement approaches.
4.3 Mood Disorders
Dysregulation of the endogenous opioid system has been implicated in the pathogenesis of mood disorders, such as depression and anxiety (Nestler & Carlezon, 2006). Depressed individuals often exhibit decreased endorphin levels and increased sensitivity to pain and stress (Younger et al., 2009). Exercise therapy and other endorphin-releasing interventions can improve mood and reduce symptoms of depression and anxiety (Craft, 2005). Exercise has been shown to increase endorphin levels in individuals with depression, improving self-esteem, sleep quality, and overall quality of life (Blumenthal et al., 1999). Furthermore, mindfulness-based practices and social support can also enhance endorphin release, promoting emotional well-being and reducing the risk of relapse (Gotlib & Hamilton, 2008).
4.4 Neurodegenerative Diseases
Emerging evidence suggests that endorphins may play a protective role in neurodegenerative diseases, such as Alzheimer’s disease and Parkinson’s disease (Martins et al., 2006). Endorphins can reduce inflammation, protect neurons from oxidative stress, and promote neurogenesis (Ghasemi et al., 2010). Exercise therapy has been shown to improve cognitive function and reduce the risk of dementia in older adults (Lautenschlager et al., 2008). Exercise may exert its neuroprotective effects by increasing endorphin levels and promoting the growth and survival of neurons (Cotman et al., 2007). Furthermore, lifestyle interventions that promote social engagement and cognitive stimulation can also enhance endorphin release, improving cognitive function and reducing the risk of neurodegenerative diseases (Fratiglioni et al., 2004). However, the exact mechanisms underlying the neuroprotective effects of endorphins require further investigation.
Many thanks to our sponsor Maggie who helped us prepare this research report.
5. Limitations and Future Directions
Despite significant advances in our understanding of endorphins, several limitations remain. First, measuring endorphin levels in the brain is technically challenging, and most studies rely on indirect measures, such as changes in pain perception or mood. Developing more sensitive and non-invasive techniques for measuring endorphin levels in the brain is crucial for advancing our understanding of their physiological roles. Second, the interactions between endorphins and other neurotransmitter systems are complex and not fully understood. Future research should focus on elucidating the cross-talk between the endogenous opioid system and other neurotransmitter systems, such as dopamine, serotonin, and endocannabinoids. Third, the therapeutic potential of endorphin-releasing interventions needs further evaluation in well-designed clinical trials. Rigorous studies are needed to determine the optimal type, intensity, and duration of exercise, acupuncture, and mindfulness-based practices for maximizing endorphin release and improving clinical outcomes. Fourth, individual differences in endorphin response should be taken into account. Factors such as genetics, age, sex, and prior exposure to stress can influence the magnitude of endorphin release in response to various stimuli. Personalized approaches that tailor interventions to individual needs and characteristics may be more effective in maximizing the therapeutic benefits of endorphins.
Many thanks to our sponsor Maggie who helped us prepare this research report.
6. Conclusion
Endorphins are endogenous opioid neuropeptides with a wide range of physiological effects, extending far beyond pain modulation and exercise-induced euphoria. They play a critical role in stress response, immune modulation, social behavior, and reward circuitry. Endorphin-releasing interventions, such as exercise, acupuncture, and mindfulness-based practices, hold promise for the management of pain, addiction, mood disorders, and neurodegenerative diseases. However, further research is needed to fully elucidate the complex roles of endorphins in health and disease and to optimize the therapeutic application of endorphin-releasing interventions. A deeper understanding of the neurobiological mechanisms and physiological effects of endorphins will undoubtedly lead to the development of novel and effective strategies for improving human health and well-being.
Many thanks to our sponsor Maggie who helped us prepare this research report.
References
Akil, H., Watson, S. J., Young, E., Lewis, M. E., Khachaturian, H., & Walker, J. M. (1984). Endogenous opioids: biology and function. Annual review of neuroscience, 7(1), 223-255.
Arborelius, L., Owens, M. J., Plotsky, P. M., & Nemeroff, C. B. (1999). The role of corticotropin-releasing factor in depression and anxiety disorders. Journal of Endocrinology, 160(1), 1-12.
Basbaum, A. I., & Fields, H. L. (1978). Endogenous pain control mechanisms: review and hypothesis. Annals of neurology, 4(6), 451-462.
Bloom, F. E. (1996). Neurotransmission and the central nervous system. In Fundamental neuroscience (pp. 245-267). Academic Press.
Blumenthal, J. A., Babyak, M. A., Moore, K. A., Craighead, W. E., Herman, S., Khatri, P., … & Sherwood, A. (1999). Effects of exercise training on older patients with major depression. Archives of internal medicine, 159(19), 2349-2356.
Bodnar, R. J. (2016). Endogenous opioids and behavior: 2015. Peptides, 83, 1-22.
Boecker, H., Sprenger, T., Spilker, M. E., Henriksen, G., Koppenhoefer, M., Wagner, K. J., … & Tolle, T. R. (2008). The runner’s high: Opioidergic mechanisms in the human brain. Cerebral Cortex, 18(11), 2523-2531.
Brown, R. A., Abrantes, A. M., Read, J. P., Marcus, B. H., Jakicic, J. M., Strong, D. R., … & Kahler, C. W. (2008). Exercise for smoking cessation: systematic review and meta-analysis. Addiction, 103(9), 1461-1469.
Carr, D. J., & Sergerstrom, H. L. (1993). Opiates and infection. Chemical immunology, 54, 185-202.
Cotman, C. W., Berchtold, N. C., & Christie, L. A. (2007). Exercise: a behavioral intervention to prevent Alzheimer’s disease. Trends in neurosciences, 30(12), 617-625.
Craft, L. L. (2005). Exercise and clinical depression: examining two psychological mechanisms. Psychology of sport and exercise, 6(2), 151-169.
Di Chiara, G. (1998). A motivational circuitry for behavioral economics. Neuroscience & Biobehavioral Reviews, 22(3), 303-328.
Fields, H. L. (2004). State-dependent opioid modulation of pain. Nature Reviews Neuroscience, 5(7), 565-575.
Fratiglioni, L., Paillard-Borg, S., & Winblad, B. (2004). An active and socially integrated lifestyle in late life is associated with a reduced risk of dementia. The Lancet Neurology, 3(6), 343-353.
Geneen, L. J., Moore, R. A., Clarke, C., Martin, D., Colvin, L. A., & Smith, B. H. (2017). Physical activity and exercise for chronic pain in adults: an overview of Cochrane Reviews. Cochrane Database of Systematic Reviews, *(4).
Ghasemi, M., Sadeghian, M., Dargahi, L., & Khodagholi, F. (2010). Roles of opioid receptors in neuroprotection/neurotoxicity. Pharmacology & Therapeutics, 128(3), 560-574.
Goldfarb, A. H., & Jamurtas, A. Z. (1997). Beta-endorphin response to exercise: an update. Sports medicine, 24(1), 8-16.
Gotlib, I. H., & Hamilton, J. P. (2008). Neuroimaging and depression: current status and future directions. Current directions in psychological science, 17(2), 119-123.
Guillemin, R., Vargo, T., Rossier, J., Minick, S., Ling, N., Rivier, C., … & Bloom, F. (1977). β-Endorphin and adrenocorticotropin are secreted concomitantly by the pituitary gland. Science, 197(4311), 1367-1369.
Han, J. S. (2004). Acupuncture and endorphins. Neuroscience Letters, 361(1-3), 258-261.
Hughes, J. (1975). Isolation of an endogenous compound from the brain with pharmacological properties similar to morphine. Brain research, 88(2), 295-308.
Insel, T. R. (2010). The challenge of translation in social neuroscience: from mouse to bedside. Neuron, 65(6), 746-748.
Kavelaars, A., Heijnen, C. J., & Geenen, R. (2000). The role of endogenous opioids in the interaction between the brain and the immune system. Brain, behavior, and immunity, 14(4), 219-227.
Kelly, J. F., Yeterian, J. D., & Bradstreet, C. C. (2011). Mutual-help organizations and addiction recovery: what do we know?. Journal of substance abuse treatment, 41(3), 266-279.
Knapp, R. J., Malatynska, E., Collins, N., Fang, L., Wang, J., Hruby, V. J., Roeske, W. R., & Yamamura, H. I. (1996). Delta opioid receptors. Life sciences, 58(3), 167-175.
Koob, G. F., & Le Moal, M. (2001). Drug addiction, dysregulation of reward, and allostasis. Neuropsychopharmacology, 24(2), 97-129.
Koob, G. F., & Volkow, N. D. (2010). Neurocircuitry of addiction. Neuropsychopharmacology, 35(1), 217-238.
Lautenschlager, N. T., Cox, K. L., Cyngler, B., Flicker, L., Foster, J. K., Greenop, K. R., … & Brodaty, H. (2008). Effect of cognitive activity and physical exercise on cognitive function in older adults: a randomised controlled trial. JAMA, 300(9), 1027-1037.
Lightman, S. L. (2008). The neuroendocrinology of stress: a historical perspective. Stress, 11(1), 1-6.
Lysle, D. T., Coussons-Read, M. E., Dowlati, Y., & Shavit, Y. (2004). Opioids and the immune system. Pain, 112(1-2), 5-9.
Mansour, A., Khachaturian, H., Lewis, M. E., Akil, H., & Watson, S. J. (1994). Anatomy of CNS opioid receptors. Trends in neurosciences, 17(7), 298-302.
Martins, R. N., Clarnette, R., Redhead, M., Hallmayer, J., Tanner, J., & Masters, C. L. (2006). A role for beta-amyloid in Alzheimer’s disease. Molecular psychiatry, 11(1), 29-45.
Mayer, D. J., Price, D. D., & Rafii, A. (1977). Antagonism of acupuncture analgesia in man by the narcotic antagonist naloxone. Brain research, 121(2), 368-372.
McEwen, B. S. (1998). Protective and damaging effects of stress mediators. New England Journal of Medicine, 338(3), 171-179.
Millan, M. J. (2002). The role of opioid receptors in stress. Dialogues in clinical neuroscience, 4(3), 281.
Moles, A., Kieffer, B. L., & D’Amato, F. R. (2004). Deficit in attachment behavior in mice lacking the μ-opioid receptor. Science, 304(5679), 1983-1986.
Nakanishi, S., Inoue, A., Kita, T., Nakamura, M., Chang, A. C., Cohen, S. N., & Numa, S. (1979). Nucleotide sequence of cloned cDNA for bovine corticotropin-β-lipotropin precursor. Nature, 278(5703), 423-427.
Nestler, E. J., & Carlezon, W. A. (2006). The mesolimbic dopamine pathway: role in depression. Biological psychiatry, 59(12), 1151-1159.
North, R. A. (1992). Opioid receptors and their function in the enteric nervous system. American Journal of Physiology-Gastrointestinal and Liver Physiology, 263(4), G581-G588.
Nummenmaa, L., Tuominen, L., Dunbar, R. I., Hietanen, J. K., & Hari, R. (2016). Social touch modulates endogenous opioid neurotransmission in humans. Proceedings of the National Academy of Sciences, 113(3), 644-649.
Ossipov, M. H., Morimura, K., & Porreca, F. (2010). Descending pain modulation and chronic pain. Pain, 148(2), 195-215.
Panksepp, J. (1998). Affective neuroscience: The foundations of human and animal emotions. Oxford university press.
Panksepp, J., & Lahvis, G. P. (2007). Social emotions: an evolutionary and neuroscientific perspective. Emotion, 7(3), 453.
Pert, C. B., & Snyder, S. H. (1973). Opiate receptor: demonstration in nervous tissue. Science, 179(4078), 1011-1014.
Petitto, J. M., Claman, H. N., Fletcher, M. A., Ironson, G., Kumar, M., McHutchison, J., … & Evans, D. L. (1991). Alterations in circulating human lymphocyte subsets associated with self-reported mood and stress. Psychosomatic medicine, 53(1), 1-8.
Reiner, K., Tibi, L., & Lipsitz, J. D. (2013). Mindfulness-based interventions for chronic pain: a systematic review. Pain medicine, 14(1), 22-37.
Satoh, M., & Minami, M. (1995). Molecular pharmacology of the opioid receptors. Pharmacology & Therapeutics, 68(3), 343-364.
Sluka, K. A., & Walsh, D. (2003). Transcutaneous electrical nerve stimulation: basic science mechanisms and clinical effectiveness. Pain, 103(2), 175-202.
Smith, A. I., & Funder, J. W. (1988). Proopiomelanocortin processing and the regulation of the hypothalamic-pituitary-adrenal axis. Endocrine reviews, 9(2), 159-179.
Vonvoigtlander, P. F., Lahti, R. A., & Ludens, J. H. (1983). U-50,488: a selective and structurally novel non-mu (kappa) opioid agonist. Journal of Pharmacology and Experimental Therapeutics, 224(1), 7-12.
Weber, R. J., Pert, A., Chrousos, G. P., Gold, P. W., & Liang, J. (1987). The corticotropin-releasing hormone and interleukin-1 beta immunoreactive cell bodies are juxtaposed in the paraventricular nucleus of the hypothalamus. Brain research, 426(2), 369-374.
Weinhold, C., Best, D., & Müller, A. (2010). Physical exercise and addiction treatment: A systematic review of controlled studies. Substance Use & Misuse, 45(9), 1339-1363.
Williams, J. T., Ingram, S. L., Henderson, G., Chavkin, C., von Zastrow, M., Whistler, J. L., & Christie, M. J. (2013). Regulation of μ-opioid receptors: desensitization, phosphorylation, internalization, and tolerance. Pharmacological reviews, 65(1), 223-254.
Wise, R. A. (2002). Brain reward circuitry: insights from unsensed incentives. Neuron, 36(2), 229-240.
Younger, J., Chuong, B., Goddard, G., & Mackey, S. (2009). The endogenous opioid system and chronic pain. Current rheumatology reports, 11(6), 474-481.
Be the first to comment