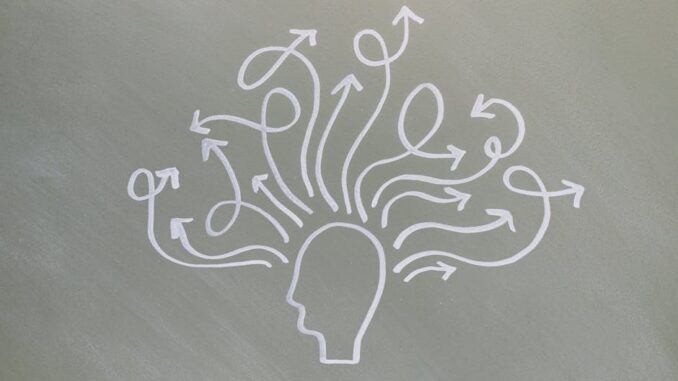
Abstract
Dopamine, a catecholamine neurotransmitter, plays a crucial role in a wide array of brain functions, extending far beyond its well-established involvement in reward and motivation. This research report provides a comprehensive overview of dopamine, exploring its synthesis, metabolism, receptor subtypes, and signaling pathways. We delve into the canonical functions of dopamine in motor control, reward processing, and cognitive functions, as well as its emerging roles in neuroendocrine regulation, sensory processing, and immune modulation. Furthermore, we examine the dysregulation of dopamine systems in various neurological and psychiatric disorders, including Parkinson’s disease, schizophrenia, addiction, and attention-deficit/hyperactivity disorder (ADHD). Finally, we discuss current and potential therapeutic strategies targeting the dopaminergic system, considering their efficacy and limitations, and highlighting the need for further research to develop more targeted and effective interventions.
Many thanks to our sponsor Maggie who helped us prepare this research report.
1. Introduction
Dopamine (3,4-dihydroxyphenethylamine) is a pivotal neurotransmitter in the central nervous system (CNS), acting as a chemical messenger mediating communication between neurons. Its synthesis from the amino acid precursor L-tyrosine, its degradation by enzymes like monoamine oxidase (MAO) and catechol-O-methyltransferase (COMT), and its interaction with a diverse family of receptors (D1-D5) contribute to its complex and multifaceted role in brain function. Initially recognized for its involvement in motor control and the pathophysiology of Parkinson’s disease, dopamine has since been implicated in a broad spectrum of processes, including reward and motivation, cognition, executive functions, learning and memory, sleep-wake cycles, and neuroendocrine regulation.
The disruption of dopamine signaling is a hallmark of several neurological and psychiatric disorders, underscoring its clinical significance. Understanding the intricacies of dopamine synthesis, release, reuptake, and receptor activation is crucial for developing effective therapeutic strategies to address these disorders. This research report aims to provide an in-depth exploration of dopamine, covering its synthesis and metabolism, receptor subtypes and signaling pathways, physiological functions, involvement in various diseases, and current and potential therapeutic approaches.
Many thanks to our sponsor Maggie who helped us prepare this research report.
2. Dopamine Synthesis, Metabolism, and Transport
2.1. Synthesis
Dopamine synthesis is a tightly regulated process initiated by the uptake of L-tyrosine into dopaminergic neurons. This uptake is facilitated by large neutral amino acid transporters (LATs). Once inside the neuron, L-tyrosine is converted to L-dihydroxyphenylalanine (L-DOPA) by the enzyme tyrosine hydroxylase (TH). TH is the rate-limiting enzyme in dopamine synthesis, and its activity is regulated by various factors, including neuronal activity, phosphorylation, and feedback inhibition by dopamine itself. L-DOPA is then rapidly decarboxylated to dopamine by aromatic L-amino acid decarboxylase (AADC), also known as DOPA decarboxylase. This enzyme is not specific to dopaminergic neurons and is also found in other tissues. The newly synthesized dopamine is then transported into synaptic vesicles by the vesicular monoamine transporter 2 (VMAT2), where it is stored and protected from degradation.
2.2. Metabolism
Dopamine metabolism primarily occurs through two enzymatic pathways: oxidative deamination by monoamine oxidase (MAO) and O-methylation by catechol-O-methyltransferase (COMT). MAO exists in two isoforms, MAO-A and MAO-B, with different substrate specificities and tissue distribution. MAO-A is primarily found in neurons and metabolizes dopamine, serotonin, and norepinephrine. MAO-B is mainly found in glial cells and metabolizes dopamine and other trace amines. COMT catalyzes the transfer of a methyl group from S-adenosylmethionine (SAM) to dopamine, producing 3-methoxytyramine (3-MT). The relative contribution of MAO and COMT to dopamine metabolism varies depending on the brain region and cellular compartment. Both enzymes generate metabolites that are further processed and eventually excreted. The major metabolites include homovanillic acid (HVA) and 3,4-dihydroxyphenylacetic acid (DOPAC), which can be measured in cerebrospinal fluid (CSF) and urine as indicators of dopamine turnover.
2.3. Transport
The dopamine transporter (DAT) is a crucial protein responsible for the reuptake of dopamine from the synaptic cleft back into the presynaptic neuron. DAT is a member of the solute carrier 6 (SLC6) family of transporters and is expressed primarily in dopaminergic neurons. It uses the electrochemical gradient of sodium and chloride ions to drive dopamine transport against its concentration gradient. DAT plays a critical role in regulating the concentration of dopamine in the synaptic cleft, thereby controlling the duration and intensity of dopaminergic signaling. DAT is also the target of several psychostimulant drugs, such as cocaine and amphetamine, which block or reverse its function, leading to increased dopamine levels in the synapse.
Many thanks to our sponsor Maggie who helped us prepare this research report.
3. Dopamine Receptors and Signaling Pathways
Dopamine exerts its diverse effects by interacting with a family of G protein-coupled receptors (GPCRs) classified into five subtypes: D1, D2, D3, D4, and D5. These receptors are further grouped into two subfamilies based on their sequence homology and signaling properties: the D1-like receptors (D1 and D5) and the D2-like receptors (D2, D3, and D4).
3.1. D1-like Receptors (D1 and D5)
D1 and D5 receptors are coupled to Gs proteins, which activate adenylyl cyclase, leading to an increase in intracellular cyclic AMP (cAMP) levels. Elevated cAMP activates protein kinase A (PKA), which phosphorylates various downstream targets, including transcription factors such as cAMP response element-binding protein (CREB). Activation of D1-like receptors generally leads to neuronal excitation and increased synaptic plasticity. D1 receptors are highly expressed in the striatum, cortex, and hippocampus, while D5 receptors have a more restricted distribution. Genetic studies have revealed subtle differences in their functional roles. For instance, D1 receptors are crucial for motor activity and reward learning, while D5 receptors may play a more prominent role in cognitive functions, particularly working memory.
3.2. D2-like Receptors (D2, D3, and D4)
D2, D3, and D4 receptors are coupled to Gi/o proteins, which inhibit adenylyl cyclase, leading to a decrease in cAMP levels. They also activate inwardly rectifying potassium (GIRK) channels and inhibit voltage-gated calcium channels. Activation of D2-like receptors generally leads to neuronal inhibition and decreased synaptic plasticity. D2 receptors exist in two isoforms, D2L (long) and D2S (short), generated by alternative splicing. D2L is the predominant isoform in the striatum and acts as a postsynaptic receptor mediating the effects of dopamine. D2S is primarily found in presynaptic terminals and acts as an autoreceptor, regulating dopamine synthesis and release. D3 receptors are highly expressed in the nucleus accumbens and other limbic regions and are implicated in reward-related behaviors and motivation. D4 receptors have a more restricted distribution and are found in the frontal cortex, amygdala, and hippocampus. They have been implicated in cognitive functions and emotional regulation.
3.3. Receptor Heteromerization
Increasing evidence suggests that dopamine receptors can form heteromers, complexes of two or more different receptor subtypes. Receptor heteromerization can alter receptor signaling properties, pharmacology, and trafficking. For example, D1-D2 receptor heteromers have been shown to exhibit unique signaling characteristics compared to individual D1 or D2 receptors. These heteromers may play a crucial role in integrating dopaminergic signaling and fine-tuning neuronal activity. Disruptions in receptor heteromerization have been implicated in various neurological and psychiatric disorders, highlighting the importance of understanding their role in dopaminergic neurotransmission.
Many thanks to our sponsor Maggie who helped us prepare this research report.
4. Physiological Functions of Dopamine
4.1. Motor Control
Dopamine plays a critical role in motor control through the nigrostriatal pathway, which projects from the substantia nigra pars compacta (SNc) to the dorsal striatum. Dopaminergic neurons in the SNc release dopamine onto striatal neurons, which are involved in the initiation and execution of voluntary movements. Degeneration of dopaminergic neurons in the SNc is the primary cause of Parkinson’s disease, characterized by motor deficits such as bradykinesia, rigidity, and tremor. Levodopa (L-DOPA), a precursor to dopamine, is the most common treatment for Parkinson’s disease, as it can be converted to dopamine in the brain, compensating for the loss of dopaminergic neurons.
4.2. Reward and Motivation
Dopamine is a key neurotransmitter in the mesolimbic pathway, which projects from the ventral tegmental area (VTA) to the nucleus accumbens (NAc). This pathway is central to reward processing, motivation, and reinforcement learning. Dopamine release in the NAc is triggered by rewarding stimuli, such as food, sex, and drugs of abuse. This dopamine release reinforces behaviors that lead to these rewards, increasing the likelihood that these behaviors will be repeated in the future. Dysregulation of the mesolimbic pathway is implicated in addiction, where compulsive drug seeking and use are driven by the overwhelming reinforcement provided by drugs of abuse.
4.3. Cognition and Executive Functions
Dopamine also plays a crucial role in cognitive functions, particularly working memory, attention, and executive functions. The mesocortical pathway, which projects from the VTA to the prefrontal cortex (PFC), is involved in these cognitive processes. Dopamine in the PFC modulates neuronal activity and synaptic plasticity, enabling the maintenance and manipulation of information in working memory, the focusing of attention, and the planning and execution of complex tasks. Deficits in dopamine signaling in the PFC are associated with cognitive impairments in schizophrenia, ADHD, and other disorders.
4.4. Neuroendocrine Regulation
Dopamine acts as a neurohormone, inhibiting the release of prolactin from the anterior pituitary gland. Dopaminergic neurons in the hypothalamus release dopamine into the hypophyseal portal system, which carries it to the pituitary gland. Dopamine binds to D2 receptors on lactotroph cells in the pituitary gland, inhibiting prolactin synthesis and secretion. Drugs that block dopamine receptors, such as antipsychotics, can lead to hyperprolactinemia, characterized by elevated prolactin levels in the blood.
4.5. Sensory Processing
Emerging evidence suggests that dopamine plays a role in sensory processing, particularly in the visual and auditory systems. Dopaminergic neurons have been identified in the retina and the cochlea, and dopamine release in these regions modulates the activity of sensory neurons. Dopamine may influence sensory perception by modulating neuronal excitability, synaptic plasticity, and signal-to-noise ratio. Dysregulation of dopamine signaling in sensory systems may contribute to sensory abnormalities in schizophrenia and other disorders.
4.6. Immune Modulation
Recent studies have revealed a bidirectional interaction between the dopaminergic system and the immune system. Immune cells, such as lymphocytes and macrophages, express dopamine receptors and can synthesize and release dopamine. Dopamine can modulate immune cell activity, affecting cytokine production, cell proliferation, and migration. Conversely, inflammatory cytokines can influence dopamine synthesis, release, and reuptake in the brain. This interaction between the dopaminergic and immune systems may play a role in the pathogenesis of neuroinflammatory disorders.
Many thanks to our sponsor Maggie who helped us prepare this research report.
5. Dopamine Dysregulation in Neurological and Psychiatric Disorders
5.1. Parkinson’s Disease
Parkinson’s disease is a neurodegenerative disorder characterized by the progressive loss of dopaminergic neurons in the substantia nigra pars compacta (SNc). This loss of dopamine leads to motor deficits, including bradykinesia, rigidity, tremor, and postural instability. The primary treatment for Parkinson’s disease is levodopa (L-DOPA), which is converted to dopamine in the brain. However, long-term L-DOPA treatment can lead to motor complications, such as dyskinesias. Other treatments for Parkinson’s disease include dopamine agonists, which directly stimulate dopamine receptors, and MAO-B inhibitors, which prevent the breakdown of dopamine. Deep brain stimulation (DBS) of the subthalamic nucleus or globus pallidus interna is also an effective treatment for some patients with Parkinson’s disease.
5.2. Schizophrenia
Schizophrenia is a chronic psychiatric disorder characterized by positive symptoms (hallucinations and delusions), negative symptoms (blunted affect and social withdrawal), and cognitive deficits. The dopamine hypothesis of schizophrenia posits that excessive dopamine activity in the mesolimbic pathway contributes to positive symptoms, while reduced dopamine activity in the mesocortical pathway contributes to negative symptoms and cognitive deficits. Antipsychotic medications, which block dopamine receptors, are the primary treatment for schizophrenia. First-generation antipsychotics (FGAs), such as haloperidol, primarily block D2 receptors and are effective in reducing positive symptoms but can cause extrapyramidal side effects (EPS). Second-generation antipsychotics (SGAs), such as clozapine and risperidone, have a lower affinity for D2 receptors and also block serotonin receptors. SGAs are generally more effective in treating negative symptoms and have a lower risk of EPS compared to FGAs.
5.3. Addiction
Addiction is a chronic relapsing disorder characterized by compulsive drug seeking and use despite negative consequences. Drugs of abuse increase dopamine levels in the nucleus accumbens (NAc), reinforcing drug-seeking behavior. Chronic drug use can lead to neuroadaptive changes in the dopaminergic system, including decreased dopamine receptor expression and reduced dopamine release. These changes contribute to the development of tolerance, dependence, and withdrawal symptoms. Treatment for addiction typically involves detoxification, behavioral therapies, and medications. Medications that can reduce cravings and prevent relapse include naltrexone (an opioid antagonist), acamprosate (a glutamate modulator), and disulfiram (an aldehyde dehydrogenase inhibitor).
5.4. Attention-Deficit/Hyperactivity Disorder (ADHD)
ADHD is a neurodevelopmental disorder characterized by inattention, hyperactivity, and impulsivity. The pathophysiology of ADHD is thought to involve deficits in dopamine and norepinephrine signaling in the prefrontal cortex (PFC). Stimulant medications, such as methylphenidate and amphetamine, increase dopamine and norepinephrine levels in the PFC, improving attention, focus, and impulse control. Non-stimulant medications, such as atomoxetine, selectively inhibit norepinephrine reuptake and are also effective in treating ADHD.
5.5 Restless Legs Syndrome (RLS)
Restless Legs Syndrome (RLS) is a neurological disorder characterized by an irresistible urge to move the legs, often accompanied by unpleasant sensations. While the exact cause of RLS is unknown, evidence suggests that dopamine dysfunction plays a significant role. Medications that increase dopamine levels or mimic dopamine’s effects, such as dopamine agonists (e.g., pramipexole, ropinirole), are often used to treat RLS.
Many thanks to our sponsor Maggie who helped us prepare this research report.
6. Therapeutic Strategies Targeting the Dopaminergic System
6.1. Dopamine Replacement Therapy
Levodopa (L-DOPA) is the mainstay of treatment for Parkinson’s disease. L-DOPA is a precursor to dopamine that can cross the blood-brain barrier and be converted to dopamine in the brain. However, long-term L-DOPA treatment can lead to motor complications, such as dyskinesias. Combining L-DOPA with a dopa decarboxylase inhibitor (carbidopa or benserazide) prevents the peripheral conversion of L-DOPA to dopamine, reducing side effects such as nausea and hypotension.
6.2. Dopamine Agonists
Dopamine agonists directly stimulate dopamine receptors and are used to treat Parkinson’s disease and RLS. Dopamine agonists include pramipexole, ropinirole, and rotigotine. These medications have a longer duration of action than L-DOPA and can be used as monotherapy in early-stage Parkinson’s disease or as adjunctive therapy in later stages.
6.3. Monoamine Oxidase Inhibitors (MAOIs)
MAOIs prevent the breakdown of dopamine by inhibiting the enzyme monoamine oxidase. MAOIs are used to treat Parkinson’s disease and depression. Selegiline and rasagiline are selective MAO-B inhibitors that are used to treat Parkinson’s disease. These medications increase dopamine levels in the brain by preventing its metabolism.
6.4. Catechol-O-Methyltransferase (COMT) Inhibitors
COMT inhibitors prevent the breakdown of dopamine by inhibiting the enzyme catechol-O-methyltransferase. COMT inhibitors, such as entacapone and tolcapone, are used as adjunctive therapy to L-DOPA in Parkinson’s disease. These medications prolong the duration of action of L-DOPA by preventing its metabolism.
6.5. Dopamine Receptor Antagonists
Dopamine receptor antagonists block dopamine receptors and are used to treat schizophrenia and other psychotic disorders. Antipsychotic medications, such as haloperidol, clozapine, and risperidone, are dopamine receptor antagonists. These medications reduce dopamine activity in the brain, alleviating positive symptoms of schizophrenia.
6.6. Dopamine Transporter (DAT) Modulators
DAT modulators affect the reuptake of dopamine by the dopamine transporter. Stimulant medications, such as methylphenidate and amphetamine, block DAT and increase dopamine levels in the synaptic cleft. These medications are used to treat ADHD and narcolepsy. However, DAT modulators also have the potential for abuse and addiction.
6.7. Deep Brain Stimulation (DBS)
DBS involves the implantation of electrodes in specific brain regions, such as the subthalamic nucleus or globus pallidus interna, and the delivery of electrical stimulation. DBS is an effective treatment for Parkinson’s disease, essential tremor, and dystonia. DBS can modulate neuronal activity and improve motor symptoms in these disorders.
6.8. Gene Therapy
Gene therapy involves the delivery of genes into cells to correct genetic defects or to express therapeutic proteins. Gene therapy approaches are being developed for Parkinson’s disease, including the delivery of genes encoding enzymes involved in dopamine synthesis or neurotrophic factors that protect dopaminergic neurons. While gene therapy is still in the early stages of development, it holds promise as a potential treatment for Parkinson’s disease.
Many thanks to our sponsor Maggie who helped us prepare this research report.
7. Future Directions and Conclusion
Dopamine remains a central focus of neuroscience research due to its diverse functions and involvement in numerous neurological and psychiatric disorders. Future research should focus on several key areas:
- Refining Dopamine Receptor Subtype Specificity: The development of more selective ligands for dopamine receptor subtypes is crucial for understanding their individual roles and for developing more targeted therapies. This includes exploring allosteric modulators that fine-tune receptor function without directly activating or blocking them.
- Investigating Dopamine Heteroreceptor Complexes: Further characterization of dopamine receptor heteromers and their signaling properties is needed to understand their role in integrating dopaminergic signaling and fine-tuning neuronal activity. Developing drugs that selectively target these heteromers could offer novel therapeutic strategies.
- Elucidating the Role of Dopamine in Non-Canonical Functions: Further research is needed to understand the role of dopamine in sensory processing, immune modulation, and other non-canonical functions. This could lead to new insights into the pathogenesis of disorders such as neuroinflammatory diseases.
- Developing Novel Drug Delivery Strategies: Novel drug delivery strategies, such as nanoparticles and viral vectors, are needed to improve the delivery of dopamine-modulating drugs to specific brain regions and to minimize side effects.
- Personalized Medicine Approaches: Personalized medicine approaches that take into account individual genetic and environmental factors are needed to optimize dopamine-based therapies for different patients.
In conclusion, dopamine is a critical neurotransmitter with a wide range of functions in the brain. Dysregulation of dopamine signaling is implicated in numerous neurological and psychiatric disorders. Current therapeutic strategies targeting the dopaminergic system have limitations, highlighting the need for further research to develop more targeted and effective interventions. Continued research into the complexities of dopamine signaling will undoubtedly lead to new insights into brain function and the development of innovative treatments for neurological and psychiatric disorders. The future of dopamine research lies in refining our understanding of its complex signaling pathways, developing more selective and targeted therapies, and applying personalized medicine approaches to optimize treatment outcomes.
Many thanks to our sponsor Maggie who helped us prepare this research report.
References
- Beaulieu, J. M., & Gainetdinov, R. R. (2011). The physiology, signaling, and pharmacology of dopamine receptors. Pharmacological Reviews, 63(1), 182-217.
- Bjorklund, A., & Dunnett, S. B. (2007). Dopamine neuron systems: a guide to their anatomy and function. Trends in Neurosciences, 30(5), 194-202.
- Bromberg-Martin, E. S., Matsumoto, M., & Hikosaka, O. (2010). Dopamine in motivational control: rewarding, aversive, and alerting. Neuron, 68(5), 815-834.
- Calipari, E. S., & Ferris, M. J. (2013). Amphetamine mechanisms and actions at the dopamine terminal revisited. Journal of Neuroscience, 33(45), 17239-17249.
- Girault, J. A., & Beaulieu, J. M. (2021). Dopamine and the pathophysiology of movement disorders. Handbook of Clinical Neurology, 178, 177-203.
- Klein, M. O., & Tobin, A. J. (2015). Common and disparate mechanisms of neurodegeneration in Parkinson’s disease and Alzheimer’s disease. Cold Spring Harbor Perspectives in Medicine, 5(2), a021821.
- Nair, S. M., Monory, K., Schwarting, R. K., & Marsicano, G. (2011). CB1 cannabinoid receptors in dopamine neurons drive reward and promote psychomotor activation. Journal of Neuroscience, 31(48), 17951-17963.
- Volkow, N. D., Wang, G. J., Fowler, J. S., Tomasi, D., & Baler, R. (2010). Addiction: decreased reward sensitivity and increased expectation sensitivity drive compulsive drug seeking. Neuron, 68(5), 759-779.
- Wise, R. A. (2004). Dopamine, learning and motivation. Nature Reviews Neuroscience, 5(6), 483-494.
- Strange, P.G. (2001). Antipsychotic drugs: relevance of actions on dopamine receptor subtypes. Pharmacological therapeutics, 91(1-2), 1-19.
- Dopamine. (2024, January 27). In Wikipedia. Retrieved from https://en.wikipedia.org/wiki/Dopamine
- Elsworth, J. D., & Roth, R. H. (2023). Neurochemistry of dopamine. Scholarpedia, 18(8), 34975.
Be the first to comment