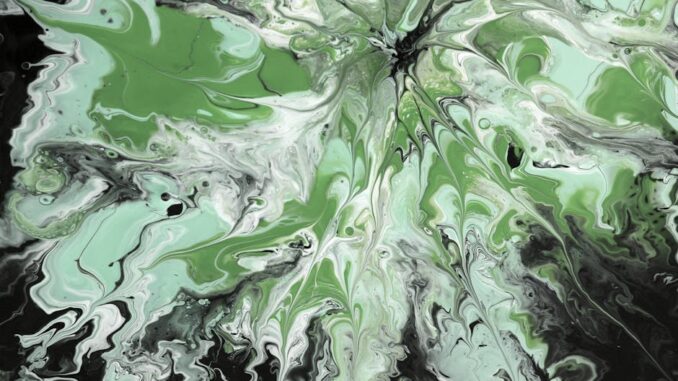
Abstract
The endocannabinoid system (ECS) is a ubiquitous and complex lipid signaling network that plays a crucial regulatory role in a diverse range of physiological processes, extending far beyond pain perception, inflammation, and immune response. This review provides a comprehensive overview of the ECS, encompassing its key components (receptors, endocannabinoids, and metabolic enzymes), its intricate interactions with other bodily systems, the multifaceted mechanisms by which cannabinoids and other agents modulate ECS activity, and the burgeoning potential of ECS-targeted therapies for a broad spectrum of disorders. We delve into the nuances of receptor subtypes, endocannabinoid biosynthesis and degradation pathways, and the emerging role of less-studied components such as the transient receptor potential vanilloid 1 (TRPV1) receptor. Furthermore, we critically examine the complexities of individual variability in ECS function arising from genetic polymorphisms, environmental factors, and disease states, and how this impacts responses to cannabinoid-based treatments and other ECS modulators. Finally, we explore the evolving landscape of ECS-based therapeutic strategies, addressing both the promise and the challenges associated with targeting this multifaceted system. The focus of this work aims to expand the horizon of ECS knowledge beyond the conventional realm of pain management, revealing its significance in disorders such as metabolic syndrome, neurodegenerative diseases, and psychiatric conditions.
Many thanks to our sponsor Maggie who helped us prepare this research report.
1. Introduction
The endocannabinoid system (ECS) has emerged as a central regulator of homeostasis, influencing a remarkable array of physiological processes throughout the body. Initially identified through the study of the effects of cannabis and its active constituents, particularly Δ9-tetrahydrocannabinol (THC), the ECS is now recognized as an endogenous signaling network involved in processes ranging from pain and inflammation to mood, appetite, sleep, and neuroprotection. Its evolutionary conservation across diverse species underscores its fundamental importance to organismal function.
The ECS is not a single entity but rather a complex interplay of several components: cannabinoid receptors (primarily CB1 and CB2), endogenous lipid signaling molecules known as endocannabinoids (e.g., anandamide (AEA) and 2-arachidonoylglycerol (2-AG)), and the enzymes responsible for their synthesis and degradation. This intricate system allows for spatially and temporally precise modulation of cellular activity, contributing to a finely tuned response to both internal and external stimuli.
While the analgesic and anti-inflammatory properties of cannabinoids have received considerable attention, the ECS’s influence extends far beyond these areas. It is deeply involved in regulating metabolic functions, including glucose homeostasis, lipid metabolism, and energy balance, highlighting its potential role in obesity and metabolic syndrome. Furthermore, the ECS is implicated in neurodegenerative diseases such as Alzheimer’s and Parkinson’s, where it appears to exert both protective and detrimental effects depending on the context. It also plays a role in the pathogenesis of psychiatric disorders, including anxiety, depression, and schizophrenia.
This review aims to provide a comprehensive overview of the ECS, delving into its key components, its interactions with other physiological systems, and the mechanisms by which cannabinoids and other agents modulate its activity. We will critically examine the therapeutic potential of ECS-targeted interventions for a wide range of disorders, while also addressing the challenges and complexities associated with targeting this system.
Many thanks to our sponsor Maggie who helped us prepare this research report.
2. Components of the Endocannabinoid System
2.1 Cannabinoid Receptors
The cannabinoid receptors, primarily CB1 and CB2, are G protein-coupled receptors (GPCRs) that mediate the effects of both endogenous and exogenous cannabinoids. These receptors are integral to the ECS’s signaling cascade, translating ligand binding into downstream cellular effects.
2.1.1 CB1 Receptor
The CB1 receptor is one of the most abundant GPCRs in the brain and is primarily responsible for the psychoactive effects of THC. It is highly expressed in brain regions associated with cognition, memory, motor control, and reward, including the cerebral cortex, hippocampus, basal ganglia, and cerebellum. However, CB1 receptors are also found in peripheral tissues, such as the liver, adipose tissue, gastrointestinal tract, and immune cells, where they play a role in regulating various physiological processes.
The activation of CB1 receptors by endocannabinoids or exogenous cannabinoids leads to the inhibition of adenylyl cyclase, resulting in a decrease in intracellular cyclic AMP (cAMP) levels. This, in turn, modulates the activity of protein kinase A (PKA) and downstream signaling pathways. CB1 receptor activation can also modulate ion channel activity, including the inhibition of voltage-gated calcium channels and the activation of potassium channels, thereby affecting neuronal excitability and neurotransmitter release. It is important to acknowledge, that signaling is not necessarily limited to cAMP, with CB1 receptors able to influence other intracellular cascades via G proteins.
2.1.2 CB2 Receptor
The CB2 receptor is primarily expressed in immune cells, including macrophages, microglia, B cells, and T cells. Its activation modulates immune cell function, suppressing inflammation and promoting immune resolution. Unlike CB1 receptors, CB2 receptors are not highly expressed in the brain under normal conditions, which has made them attractive therapeutic targets for conditions where reducing inflammation in the brain is desirable without unwanted psychotropic effects. However, evidence suggests that CB2 receptor expression in the brain can be upregulated under pathological conditions, such as neuroinflammation, suggesting a role for these receptors in modulating neuroinflammatory processes. It is worth noting that some studies are investigating whether the CB2 receptor may interact and form heteromers with other receptors, particularly in conditions of chronic pain.
The signaling pathways activated by CB2 receptor stimulation are similar to those activated by CB1 receptors, including the inhibition of adenylyl cyclase and the modulation of ion channel activity. However, CB2 receptor activation can also activate other signaling pathways, such as the mitogen-activated protein kinase (MAPK) pathway, which is involved in cell growth, differentiation, and survival.
2.1.3 Other Receptors
Beyond CB1 and CB2, other receptors are known to interact with endocannabinoids and contribute to the overall effects of the ECS. The transient receptor potential vanilloid 1 (TRPV1) receptor, a non-selective cation channel, is activated by a variety of stimuli, including heat, capsaicin, and anandamide. TRPV1 receptor activation can contribute to pain perception, inflammation, and neuroinflammation. The G protein-coupled receptor 55 (GPR55) has also been proposed as a cannabinoid receptor, although its ligand selectivity and signaling pathways are still under investigation. GPR55 activation has been implicated in a variety of processes, including bone remodeling, cancer progression, and neuroinflammation. Further research continues to refine the understanding of receptor interaction within the ECS.
2.2 Endocannabinoids
Endocannabinoids are endogenous lipid signaling molecules that bind to cannabinoid receptors and modulate their activity. The two most well-studied endocannabinoids are anandamide (AEA) and 2-arachidonoylglycerol (2-AG).
2.2.1 Anandamide (AEA)
Anandamide (AEA), derived from the Sanskrit word “ananda” meaning bliss, was the first endocannabinoid to be discovered. It is synthesized from N-arachidonoyl phosphatidylethanolamine (NAPE) through a series of enzymatic reactions. AEA primarily binds to CB1 receptors, although it can also activate TRPV1 receptors. Its effects are relatively short-lived due to its rapid degradation by the enzyme fatty acid amide hydrolase (FAAH).
AEA has been implicated in a variety of physiological processes, including pain modulation, mood regulation, appetite control, and neuroprotection. Studies have shown that AEA levels are altered in various disease states, including anxiety, depression, and neurodegenerative disorders.
2.2.2 2-Arachidonoylglycerol (2-AG)
2-Arachidonoylglycerol (2-AG) is the most abundant endocannabinoid in the brain. It is synthesized from phosphatidylinositol through the action of phospholipase C (PLC) and diacylglycerol lipase (DAGL). 2-AG binds to both CB1 and CB2 receptors, with a higher affinity for CB2 receptors than AEA. It is primarily degraded by the enzyme monoacylglycerol lipase (MAGL).
2-AG plays a crucial role in synaptic plasticity, neuroprotection, and immune regulation. It has been shown to be involved in long-term potentiation (LTP) and long-term depression (LTD), processes that are essential for learning and memory. 2-AG also exerts neuroprotective effects by reducing excitotoxicity and oxidative stress. It is also an important modulator of immune function.
2.2.3 Other Endocannabinoids
In addition to AEA and 2-AG, other lipid molecules have been identified as potential endocannabinoids. These include N-arachidonoyldopamine (NADA), virodhamine, and noladin ether. These molecules may interact with cannabinoid receptors and other receptors, such as TRPV1, contributing to the overall effects of the ECS. Further research is needed to fully elucidate the role of these less-studied endocannabinoids.
2.3 Metabolic Enzymes
The synthesis and degradation of endocannabinoids are tightly regulated by a variety of enzymes. These enzymes control the levels of endocannabinoids in the body and influence the duration and intensity of their effects. The key enzymes involved in endocannabinoid metabolism include fatty acid amide hydrolase (FAAH), monoacylglycerol lipase (MAGL), N-acyl phosphatidylethanolamine phospholipase D (NAPE-PLD), and diacylglycerol lipase (DAGL).
2.3.1 Fatty Acid Amide Hydrolase (FAAH)
Fatty acid amide hydrolase (FAAH) is the primary enzyme responsible for the degradation of AEA. It hydrolyzes AEA into arachidonic acid and ethanolamine, thereby terminating its signaling. FAAH is widely distributed throughout the body, including the brain, liver, and immune cells. Inhibition of FAAH leads to an increase in AEA levels and a prolongation of its effects. FAAH inhibitors have shown promise as therapeutic agents for anxiety, pain, and inflammation. However, it has been observed that FAAH inhibitors often produce a variety of off-target effects, which can sometimes cause more harm than benefit.
2.3.2 Monoacylglycerol Lipase (MAGL)
Monoacylglycerol lipase (MAGL) is the primary enzyme responsible for the degradation of 2-AG. It hydrolyzes 2-AG into arachidonic acid and glycerol. MAGL is highly expressed in the brain and is thought to play a critical role in regulating synaptic transmission. Inhibition of MAGL leads to an increase in 2-AG levels and a prolongation of its effects. MAGL inhibitors have shown promise as therapeutic agents for neurodegenerative diseases, pain, and inflammation. However, some studies suggest that increasing 2-AG levels may have both beneficial and detrimental effects, depending on the context.
2.3.3 N-Acyl Phosphatidylethanolamine Phospholipase D (NAPE-PLD)
N-acyl phosphatidylethanolamine phospholipase D (NAPE-PLD) is the enzyme responsible for the synthesis of NAPE, the precursor to AEA. It cleaves NAPE to produce AEA and phosphatidic acid. NAPE-PLD is expressed in a variety of tissues, including the brain, liver, and immune cells. Regulation of NAPE-PLD activity is thought to be important for controlling AEA levels in the body.
2.3.4 Diacylglycerol Lipase (DAGL)
Diacylglycerol lipase (DAGL) is the enzyme responsible for the synthesis of 2-AG. There are two isoforms of DAGL, DAGLα and DAGLβ, which are differentially expressed in the brain. DAGLα is primarily located postsynaptically and is thought to be involved in the synthesis of 2-AG that acts as a retrograde messenger. DAGLβ is primarily located presynaptically and is thought to be involved in the synthesis of 2-AG that regulates presynaptic function. Modulation of DAGL activity may offer therapeutic potential for neurological and psychiatric disorders.
Many thanks to our sponsor Maggie who helped us prepare this research report.
3. Interaction of the ECS with Other Bodily Systems
The ECS does not operate in isolation; it interacts extensively with other physiological systems, including the nervous, immune, endocrine, and cardiovascular systems. These interactions are crucial for maintaining homeostasis and responding to various stressors.
3.1 ECS and the Nervous System
The ECS plays a pivotal role in regulating neuronal excitability, synaptic plasticity, and neurotransmitter release. Endocannabinoids act as retrograde messengers, traveling from the postsynaptic neuron to the presynaptic neuron to modulate neurotransmitter release. This retrograde signaling mechanism is crucial for synaptic plasticity, which is essential for learning and memory. Activation of CB1 receptors on presynaptic neurons inhibits the release of both excitatory and inhibitory neurotransmitters, allowing for fine-tuned modulation of neuronal activity. Furthermore, the ECS interacts with other neurotransmitter systems, such as the dopamine, serotonin, and glutamate systems, influencing mood, motivation, and cognitive function. It can be argued that understanding the ECS’s modulatory effects can aid in the management of mood disorders.
3.2 ECS and the Immune System
The ECS plays a critical role in regulating immune function and inflammation. CB2 receptors are highly expressed on immune cells, and their activation suppresses inflammatory responses and promotes immune resolution. Endocannabinoids can modulate the production of cytokines and chemokines, which are important mediators of inflammation. Furthermore, the ECS interacts with other immune signaling pathways, such as the NF-κB pathway, influencing the activation and differentiation of immune cells. Dysregulation of the ECS has been implicated in various autoimmune and inflammatory diseases, suggesting that targeting the ECS may offer therapeutic potential for these conditions. It is worth noting that excessive or chronic activation of the ECS might also have an immunosuppressive effect.
3.3 ECS and the Endocrine System
The ECS interacts with the endocrine system to regulate hormone release and metabolism. CB1 receptors are expressed in the hypothalamus and pituitary gland, which are key regulators of hormone secretion. Activation of CB1 receptors can modulate the release of hormones such as cortisol, insulin, and growth hormone. Furthermore, the ECS influences the activity of the hypothalamic-pituitary-adrenal (HPA) axis, which is the body’s primary stress response system. Dysregulation of the ECS has been implicated in various endocrine disorders, such as obesity, diabetes, and adrenal insufficiency. The role that the ECS plays in hunger regulation is an area under ongoing research.
3.4 ECS and the Cardiovascular System
The ECS influences cardiovascular function through both central and peripheral mechanisms. CB1 receptors are expressed in the brainstem regions that regulate blood pressure and heart rate. Activation of CB1 receptors in these regions can decrease blood pressure and heart rate. However, CB1 receptors are also expressed in peripheral blood vessels, and their activation can lead to vasodilation or vasoconstriction, depending on the context. CB2 receptors are expressed in immune cells that infiltrate atherosclerotic plaques, and their activation can reduce inflammation and promote plaque stability. Dysregulation of the ECS has been implicated in various cardiovascular diseases, such as hypertension, atherosclerosis, and heart failure.
Many thanks to our sponsor Maggie who helped us prepare this research report.
4. Mechanisms of Cannabinoid Modulation of the ECS
Cannabinoids, both endogenous (endocannabinoids) and exogenous (phytocannabinoids and synthetic cannabinoids), modulate the activity of the ECS through a variety of mechanisms. These mechanisms include direct activation or inhibition of cannabinoid receptors, modulation of endocannabinoid synthesis and degradation, and interactions with other signaling pathways.
4.1 Direct Receptor Activation
Many cannabinoids, such as THC and cannabidiol (CBD), directly bind to and activate or inhibit cannabinoid receptors. THC is a partial agonist at both CB1 and CB2 receptors, meaning that it binds to these receptors and activates them to a certain extent. This activation leads to a cascade of downstream signaling events, resulting in the various effects associated with THC, including psychoactivity, analgesia, and immunosuppression. CBD, on the other hand, has a low affinity for both CB1 and CB2 receptors and is thought to exert its effects through other mechanisms, such as modulation of endocannabinoid synthesis and degradation, and interactions with other receptors, such as TRPV1.
4.2 Modulation of Endocannabinoid Synthesis and Degradation
Cannabinoids can also modulate the activity of the ECS by influencing the synthesis and degradation of endocannabinoids. For example, CBD has been shown to inhibit FAAH, the enzyme responsible for the degradation of AEA. This inhibition leads to an increase in AEA levels and a prolongation of its effects. Similarly, some cannabinoids can inhibit MAGL, the enzyme responsible for the degradation of 2-AG, leading to an increase in 2-AG levels. By modulating endocannabinoid synthesis and degradation, cannabinoids can fine-tune the activity of the ECS and influence a variety of physiological processes. The mechanism by which CBD interacts with these enzymes is still up for debate, with some suggesting that it is not a direct inhibitor.
4.3 Interactions with Other Signaling Pathways
Cannabinoids can also interact with other signaling pathways, such as the opioid, serotonin, and dopamine systems, to modulate their effects. For example, THC has been shown to enhance the release of dopamine in the brain, contributing to its rewarding and addictive properties. CBD has been shown to interact with the serotonin system, influencing mood and anxiety. Furthermore, cannabinoids can interact with the opioid system to modulate pain perception. These interactions with other signaling pathways highlight the complex and multifaceted nature of cannabinoid action.
Many thanks to our sponsor Maggie who helped us prepare this research report.
5. Individual Variability in ECS Function and Response to Cannabis-Based Treatments
Individual variability in ECS function plays a significant role in determining the response to cannabis-based treatments. This variability arises from a combination of genetic, environmental, and lifestyle factors.
5.1 Genetic Polymorphisms
Genetic polymorphisms in genes encoding cannabinoid receptors, metabolic enzymes, and other ECS components can influence individual differences in ECS function. For example, polymorphisms in the CNR1 gene, which encodes the CB1 receptor, have been associated with variations in pain sensitivity, anxiety, and addiction risk. Polymorphisms in the FAAH gene have been associated with variations in AEA levels and sensitivity to the effects of cannabis. These genetic polymorphisms can influence an individual’s response to cannabis-based treatments and may explain why some individuals experience more pronounced effects than others.
5.2 Environmental and Lifestyle Factors
Environmental and lifestyle factors, such as diet, stress, and exercise, can also influence ECS function. For example, a diet high in omega-6 fatty acids can increase the production of endocannabinoids, whereas a diet high in omega-3 fatty acids can reduce endocannabinoid production. Chronic stress can alter ECS function, leading to changes in anxiety, mood, and pain sensitivity. Exercise has been shown to increase endocannabinoid levels in the brain, contributing to the “runner’s high.” These environmental and lifestyle factors can interact with genetic predispositions to influence individual variability in ECS function and response to cannabis-based treatments.
5.3 Disease States
Disease states can also affect ECS function. For example, neuroinflammatory conditions, such as multiple sclerosis and Alzheimer’s disease, can lead to an upregulation of CB2 receptors in the brain. Chronic pain conditions, such as fibromyalgia and neuropathic pain, can alter endocannabinoid levels and receptor sensitivity. Psychiatric disorders, such as anxiety, depression, and schizophrenia, have been associated with dysregulation of the ECS. These disease-related changes in ECS function can influence the response to cannabis-based treatments and may require tailored therapeutic approaches.
Many thanks to our sponsor Maggie who helped us prepare this research report.
6. Therapeutic Potential of ECS-Targeted Therapies
The ECS has emerged as a promising therapeutic target for a wide range of disorders. ECS-targeted therapies include cannabinoid receptor agonists and antagonists, inhibitors of endocannabinoid metabolism, and agents that modulate ECS activity indirectly.
6.1 Pain Management
Cannabinoids have been used for centuries to manage pain. THC is an effective analgesic for various types of pain, including neuropathic pain, inflammatory pain, and cancer pain. However, its psychoactive effects can limit its use in some patients. CBD has also shown promise as an analgesic, particularly for inflammatory pain and neuropathic pain. Unlike THC, CBD is non-psychoactive and has a better safety profile. FAAH inhibitors have also shown promise as analgesics, particularly for chronic pain conditions. However, some FAAH inhibitors have been associated with adverse effects, such as anxiety and depression.
6.2 Inflammation and Autoimmune Diseases
The ECS plays a critical role in regulating inflammation and immune function. CB2 receptor agonists and endocannabinoids have been shown to reduce inflammation and suppress immune responses in various preclinical models of autoimmune diseases, such as multiple sclerosis, rheumatoid arthritis, and inflammatory bowel disease. CBD has also demonstrated anti-inflammatory and immunomodulatory effects in various experimental models. ECS-targeted therapies may offer a novel approach for treating inflammatory and autoimmune diseases. It is important to address that excessive or chronic activation of the ECS might also have an immunosuppressive effect, which may reduce the benefits of the immune system.
6.3 Neurological and Psychiatric Disorders
The ECS has been implicated in the pathogenesis of various neurological and psychiatric disorders, including Alzheimer’s disease, Parkinson’s disease, anxiety, depression, and schizophrenia. Cannabinoids and ECS modulators have shown promise as therapeutic agents for these conditions. CBD has demonstrated neuroprotective, anti-anxiety, and antidepressant effects in various preclinical and clinical studies. FAAH inhibitors have also shown promise as anti-anxiety and antidepressant agents. However, further research is needed to fully elucidate the therapeutic potential of ECS-targeted therapies for neurological and psychiatric disorders.
6.4 Metabolic Disorders
The ECS plays a critical role in regulating metabolism and energy balance. CB1 receptor antagonists have been shown to reduce appetite and promote weight loss in obese individuals. However, CB1 receptor antagonists have also been associated with adverse psychiatric effects, limiting their clinical use. Other ECS-targeted therapies, such as inhibitors of endocannabinoid synthesis, may offer a safer approach for treating metabolic disorders. There are suggestions that CBD could promote a process known as “browning” of adipose tissue.
Many thanks to our sponsor Maggie who helped us prepare this research report.
7. Challenges and Future Directions
Despite the promising therapeutic potential of ECS-targeted therapies, several challenges remain.
7.1 Off-Target Effects
Many cannabinoids and ECS modulators have off-target effects, which can limit their clinical use. For example, THC can cause psychoactive effects, anxiety, and paranoia. CBD can interact with other drugs, potentially leading to adverse effects. FAAH inhibitors have been associated with anxiety, depression, and cognitive impairment. Developing more selective and targeted ECS modulators is crucial for minimizing off-target effects and maximizing therapeutic efficacy.
7.2 Individual Variability
Individual variability in ECS function can influence the response to cannabis-based treatments. Genetic polymorphisms, environmental factors, and disease states can all contribute to this variability. Tailoring ECS-targeted therapies to individual needs and characteristics may improve therapeutic outcomes. There is a growing consensus, that there should be a much better understanding of personalised ECS and the impact of cannabinoids on its function.
7.3 Long-Term Effects
The long-term effects of chronic cannabinoid use and ECS modulation are not fully understood. Chronic THC use has been associated with cognitive impairment, addiction, and psychiatric disorders. Chronic CBD use appears to be relatively safe, but more long-term studies are needed. It is crucial to conduct long-term studies to assess the safety and efficacy of ECS-targeted therapies.
7.4 Further Research
Future research should focus on elucidating the complex interactions between the ECS and other physiological systems, identifying novel ECS components and signaling pathways, and developing more selective and targeted ECS modulators. Furthermore, clinical trials are needed to evaluate the efficacy and safety of ECS-targeted therapies for a wide range of disorders.
Many thanks to our sponsor Maggie who helped us prepare this research report.
8. Conclusion
The endocannabinoid system (ECS) is a complex and multifaceted lipid signaling network that plays a crucial regulatory role in a diverse range of physiological processes. The ECS holds tremendous promise as a therapeutic target for a wide range of disorders, including pain, inflammation, neurological and psychiatric disorders, and metabolic disorders. However, several challenges remain, including off-target effects, individual variability, and limited understanding of long-term effects. Further research is needed to fully elucidate the therapeutic potential of ECS-targeted therapies and to develop safer and more effective treatments for a wide range of conditions.
Many thanks to our sponsor Maggie who helped us prepare this research report.
References
- Lu, H. C., & Mackie, K. (2016). An Introduction to the Endocannabinoid System. Biological psychiatry, 79(7), 516–525.
- Di Marzo, V., Piscitelli, F. (2015). The Endocannabinoid System and its Clinical Implications. Gut, 64(2), 347-358.
- Zou, S., & Kumar, U. (2018). Cannabinoid Receptors and the Endocannabinoid System: Signaling and Function in the Central Nervous System. International journal of molecular sciences, 19(3), 833.
- Cristino, L., Bisogno, T., & Di Marzo, V. (2004). Anandamide: a genuine endogenous cannabinoid. Pharmacology & therapeutics, 104(2), 85–104.
- Mechoulam, R., Hanuš, L. O., & Pertwee, R. G. (1998). Early phytocannabinoid chemistry to endocannabinoids and beyond. Nature reviews. Neuroscience, 1(2), 157–167.
- Pertwee, R. G. (2008). The diverse CB1 and CB2 receptor pharmacology of three plant cannabinoids: delta9-tetrahydrocannabinol, cannabidiol and delta9-tetrahydrocannabivarin. British journal of pharmacology, 153(2), 199–215.
- Leweke, F. M., Piomelli, D., Pahlisch, F., Muhle, C., Koethe, D., Gerth, C. W., … & Emrich, H. M. (2012). Cannabidiol induces sustained reduction of psychotic symptoms and cognitive deficits in patients with schizophrenia. Translational Psychiatry, 2(3), e94.
- McPartland, J. M., Duncan, M., Di Marzo, V., & Pertwee, R. G. (2015). Are cannabidiol and Δ(9) -tetrahydrocannabivarin negative modulators of the endocannabinoid system? A systematic review. British journal of pharmacology, 172(3), 737–753.
- Hillard, C. J. (2018). Stress, anxiety, and the endocannabinoid system: Modulation of the hypothalamic-pituitary-adrenal (HPA) axis. Cannabis and cannabinoid research, 3(1), 247–269.
- Mackie, K. (2008). Cannabinoid receptors: where they are and what they do. Journal of neuroendocrinology, 20(s1), 10–14.
- Watanabe, M., Katayama, T., Iwamura, T., & Yamaoka, M. (2007). Molecular and functional identification of cannabinoid CB2 receptors in human astrocytes. Neuroscience letters, 420(2), 99–104.
- Rice, A. S. C., & Patel, S. (2017). Cannabinoids and pain. British journal of anaesthesia, 119(1), 98–108.
- Maroon, J., & Bost, J. (2018). Review of the neurological benefits of phytocannabinoids. Surgical neurology international, 9, 91.
- Nagarkatti, P., Pandey, R., Rieder, S. A., Hegde, V. L., & Nagarkatti, M. (2009). Cannabinoids as novel anti-inflammatory drugs. Future medicinal chemistry, 1(7), 1333–1349.
- Pacher, P., Bátkai, S., & Kunos, G. (2006). The endocannabinoid system as an emerging target of pharmacotherapy. Pharmacological reviews, 58(3), 389–462.
- Campos, A. C., Moreira, F. A., Gomes, F. V., Del Bel, E. A., & Guimarães, F. S. (2012). Multiple mechanisms involved in the large-spectrum therapeutic potential of cannabidiol in psychiatric disorders. Philosophical Transactions of the Royal Society B: Biological Sciences, 367(1607), 3364–3378.
- Piomelli, D. (2013). The endocannabinoid system: a drug discovery perspective. Current opinion in pharmacology, 13(1), 52–59.
- Ligresti, A., De Petrocellis, L., & Di Marzo, V. (2016). From Phytocannabinoids to Endocannabinoids and Back: A Promiscuous System. Nature Reviews Neuroscience, 17(8), 501-518.
- Russo, E. B. (2019). The Case for the Entourage Effect and Conventional Breeding of Clinical Cannabis: No “Strain,” No Gain. Frontiers in Plant Science, 10, 149.
- Finan, J. B., Phillips, S. A., Lintzeris, N., Swift, W., Galettis, C., McGregor, I. S., … & Arnold, J. C. (2013). Self-reported cannabis effects and individual differences: a focus on sensitivity to anxiety, paranoia and psychosis. PloS one, 8(5), e63423.
Be the first to comment